LEDs Come to the Forefront of General Lighting Applications
High-brightness LEDs used in solid-state lighting are changing the way light is used to define areas, shape living spaces, save energy, influence moods, improve security, and transfer information. Lighting designers using LEDs will have the ability to transform the way we see the world.
by Christopher Eichelberger
AS the general-lighting landscape continues to develop, light-emitting diodes (LEDs) are being adopted as a viable alternative to conventional lighting due to their ability to direct light and save energy. They are increasingly being used in solid-state lighting for both indoor and outdoor applica-tions, including restaurants, hotels, stores, malls, museums, offices, and residences, as well as walkways, bridges, and parking garages. From accent lighting to practical full-scale outdoor illumination, LEDs are fast becoming the light source of choice for many designers.
The benefits of LEDs, in addition to energy savings, include long life and low maintenance, dynamic lighting effects, high reliability, and near-limitless design options including color accents and color mixing. Additionally, LEDs are "green" products in that they do not contain substances that are currently known to harm the environment.
Luminous Efficacy
Compared with conventional light sources such as incandescent and halogen lamps, LEDs offer a new level of efficiency. Whereas incandescent lamps achieve ~10 lm/W, and halogen lamps around 20 lm/W, the efficacy of white LEDs is typically between 50 and 100 lm/W (depending on the type). Power consumption is low, ranging from 0.1 to 15 W, with 1 W being the most common, so even small LEDs can be used to produce high levels of light. And today's typical efficacies will quickly become history, as efficacies exceeding 100 lm/W have already been achieved in the cold white spectrum, while laboratory prototypes have been developed with efficacies of more than 185 lm/W.
An incandescent lamp converts only 3% of the input power into light, but LEDs currently convert about 20%, and that number will continue to increase as the technology advances. This enables LED light sources to provide high energy efficiency, with LED components today delivering ~ 80–100 lm/W with a projected efficacy of 188 lm/W by 2015 (Table 1). These improvements in efficiency (in terms of both lm/W and lm/$) make LEDs an attractive alternative to conventional light sources.
Table 1: LED Metrics Roadmap: the 2009 LED device prices are based on the best reported values for commercially available devices and, as shown, will decrease dramatically as LED efficacy increases over the next 5 years. Source: U.S. Dept. of Energy.
Metric |
Unit |
2009 |
2010 |
2012 |
2015 |
LED Efficacy (2800–3500K, >=85 CRI) |
lm/W |
83 |
97 |
114 |
138 |
LED Price (2800–3500K; 350 mA) |
$/klm |
46 |
25 |
11 |
4 |
LED Efficacy (4100–6500K, 70–80 CRI) |
lm/W |
132 |
147 |
164 |
188 |
LED Price (4100–6500K; 350 mA) |
$/klm |
25 |
13 |
6 |
2 |
OEM Lamp Price |
$/klm |
130 |
101 |
61 |
28 |
Challenges Facing LEDs
There are, of course, some challenges ahead. Any company looking to service the market for general lighting must have close control over performance and costs. This involves the relationship between light output (lumens) and procurement and operating costs. It also means that the cost of producing LEDs needs to be reduced over time. The upfront cost of LEDs (compared to many other types of lighting) influences payback time and the total cost of ownership (TCO). TCO and payback time are continually improving as overall efficiencies improve. Also, the relationship between light output and power consumption needs to be improved. LEDs in the future will differ from their 2009 counterparts in terms of higher current-carrying capacity, greater standardization, and a larger number of application-oriented products.
The good news is that prices for LEDs have fallen over the past 10 years by a factor of 10, and this trend is continuing at a rapid pace. With a dramatic anticipated increase in efficacy at the component level by 2015 and a corresponding reduction in price from the present levels for LEDs and for OEM luminaires as shown in Table 1, warm white (2800–3500K, with a color-rendering index (CRI) greater than or equal to 85) and cool white (4100–6500K, with a 70-80 CRI) LEDs will become the light source of choice.1
Today's high-brightness LEDs provide highly efficient directional light and greatly increased efficiency. Figure 1 shows the results being achieved in the laboratory that point the way to increased efficiency in the near future.
Energy Efficiency
Replacing existing installations today with the best available LED alternatives would save 30% of the energy going to lighting, according to the ELC (European Lamp Companies) Federation. In the future, combining LEDs, sensors, and embedded software in ambient intelligent lighting networks has the potential to save an additional 30% by 2030.
For example, a bulb-shaped LED lamp with a screw base containing six OSRAM Golden DRAGON LEDs can fully replace a 40-W incandescent lamp, producing the same amount of light as the incandescent lamp and consuming only about 8 W of power, achieving an efficiency of approximately 43 lm/W.2
Controlling Color
LEDs provide excellent color rendering and uniformity of light, i.e., a higher quality of light. As semiconductor products, white LEDs are sorted in a process known as binning [see Figs. 2(b) and 2(c)] so that customers get precisely the color locations and brightness they need for their particular applications.
LEDs emit light in saturated colors and offer a greater variety of colors than has ever been possible before. "Saturated" colors have a narrow spectral bandwidth and are found along the edges of the CIE 1931 chromaticity diagram. Chip technology (InGaN vs. InGaAlP) and doping are responsible for these colors. Specialty colors ("color on demand") can also be created using phosphor blends. These have a broader spectral bandwidth, but still have a strong spike.
All nuances of color are possible. Solid-state-lighting (SSL) systems provide the ability to tune the chromaticity of light emitted from a luminaire, depending on the application. Similarly, a wide variety of colors, not limited to the Planckian locus, can be created viamanipulation of red, blue, and green LEDs within a luminaire. This manipulation of color temperature and/or chromaticity can be accomplished via pulse width or linear modulation of the separate (warm and cool, or red, blue, and green) LED currents. (Note: The Planckian locus or curve is the path or locus that the color of an incandescent black body would take in a particular chromaticity space as the blackbody temperature changes. It describes a physical phenomenon that defines how people perceive colors as familiar and agreeable.)
Regulatory Compliance
An important part of the overall LED-lighting picture involves metrics. New technologies require new standards and testing benchmarks. From an industry perspective, standards and regulations provide a platform for a consistent language with regard to definitions, test methods, and laboratory accreditation; as well as for product design, manufacturing, and testing. From a governmental perspective, regulation helps ensure public safety, provides consumer protection, regulates energy consumption, and monitors environmental issues.
Initial standards established in 2008 are being used as guidelines for programs such as Energy Star. Over the past few years, several new standards have been issued and numerous others are currently in the developmental stage. Since 2008, the industry has seen the publication of ANSI C78.377 addressing chromaticity, LM-79 addressing luminous flux, and LM-80 covering lumen maintenance.
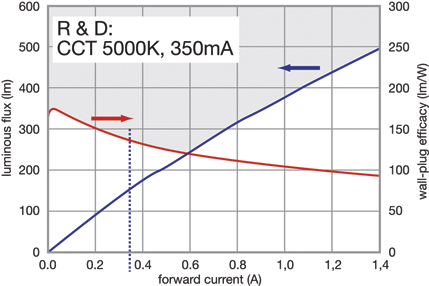
Fig. 1: At a correlated color temperature (CCT) of 5000K and a forward current of 350 mA, LEDs now under development achieve a luminous flux (blue line) of approximately 140 lm with a wall-plug efficacy (orange line) of approximately 135 lm/W.
Energy Star, a joint program of the U.S. Department of Energy and the U.S. Environmental Protection Agency, is the most recognizable symbol of energy-efficient products in the U.S. Energy-Star-qualified products are supposed to use less energy, save money, and help protect the environment. The program is now focusing on lighting applications and products that have advanced to a point where performance is equal to or better than conventional lighting technologies, based on light output, luminaire efficacy, and cost.3
Early in 2008, the American National Standard Lighting Group (ANSLG) published ANSI_NEMA_ANSLG C78.377-2008, entitled "Specifications for the Chromaticity of Solid State Lighting Products." This standard specifies the range of chromaticities recommended for general indoor lighting with SSL products, and ensures that the white-light chromaticity of the products can be communicated to consumers. It applies to LED-based SSL products with control electronics and heat sinks incorporated.
The ANSI chromaticity specification, as shown in Fig, 2(a), has been incorporated as the color standard for the Energy Star LED classification. Its goals are that it be as consistent as possible with existing fluorescent-lamp standards and reflect the current (and near future) state of SSL technology and color binning capabilities. Each of the eight quadrangles overlaps the six current ANSI seven-step MacAdam ellipses (consistent with the current Energy Star lighting criteria). Each quadrangle is defined by the range of correlated color temperature (CCT) and the distance from the Planckian locus on the chromaticity diagram.
LED manufacturers have altered their binning schemes to accommodate this ANSI standard for SSL products. For example, Fig. 2(b) shows how OSRAM's white fine binning (defined by the orange lines) is segmented to correspond with the ANSI quadrangles (as defined by the black lines).
Figure 2(c) shows how white fine binning accommodates applications in which precise color matching is critical. Each bin fits inside a three-step MacAdam ellipse, representing the limit of color difference that can be perceived by the human eye.
(a)
(b)
(c)
Fig. 2: (a) The American National Standards Institute's chromaticity specification is based on the CIE 1931 XYZ color space created by the International Commission on Illumination (CIE) in 1931. (b) White fine binning of LEDs (defined by the orange lines) is segmented to correspond with the ANSI quadrangles (as defined by the black lines). (c) White fine binning accommodates applications in which precise color matching is critical.
In June 2008, the Illuminating Engineering Society of North America (IESNA) published a documentary standard LM-79 entitled "Electrical and Photometric Measurements of Solid-State-Lighting Products." This standard describes the prescribed methods for testing the photometric characteristics of SSL products (luminaires) such as total luminous flux (lumens), luminous efficacy (lm/W), luminous-intensity distribution (candelas), chromaticity coordinates, CCT, and CRI.
The more recent LM-80 standard describes the measurement of lumen maintenance of LED light sources as well as LED packages, modules, and arrays (but not luminaires). LM-80 outlines the approved method for measuring lumen depreciation of solid-state (LED) light sources, arrays, and modules.4
Underwriters Laboratories is currently developing a safety standard for "Light-Emitting Diode (LED) Light Sources for Use in Lighting Products," which will be designated UL standard 8750. Currently, UL has an "Outline of Investigation" in place (also numbered 8750) that references all existing UL standards applicable to LED-lighting products. The purpose of the outline is to provide a comprehensive approach and listing of applicable standards for UL treatment of lighting products based on LEDs. The outline will be used until the full LED-specific document is completed.5
The LED Package
How LEDs are packaged is critical to performance. An important factor here is the beam characteristic from the LED package and, ultimately, the size of the LED. An optimized package will lead to performance gains and cost savings. For example, an LED that can offer top efficiency at higher currents up to 1 A will enable cost-efficient resource-saving designs that require not only fewer LEDs but also fewer and smaller peripheral components (ideal for "green" lighting solutions). Optics can also be integrated into the LED package, which optimizes coupling into secondary optics and, in some applications, eliminates the need for secondary optics completely.
Use of a ceramic package (as opposed to a plastic one) has improved the thermal management properties of SSL LEDs, and manufacturers are continuing to shrink the package size. Figure 3 shows ceramic LED packages from three manufacturers that illustrate today's dramatic improvements in footprint size.
Development of new chip architectures in conjunction with improved epitaxial growth technology, improvements in the phosphor converter, and high-efficiency packaging are already yielding laboratory prototypes of warm white LEDs with a chip surface of only 1 mm2 that combine a color temperature of 3000K, a CRI of 82, and an efficiency of 104 lm/W.
Indoor Lighting
LEDs are available today that are reliably efficient at high current and meet the required standards for general lighting usage in a wide range of indoor applications, including "architainment," hospitality, shop, office, industrial, and residential. LEDs are increasingly being used for special applications with demanding requirements in terms of efficiency and lifetime, such as lighting of freezer cases in supermarkets, for example. LEDs can be used to create shopping experiences designed to stimulate customers with a better accentuation of products on display. In offices, LEDs can be used to create lighting adaptable to individual needs, enhancing worker concentration and increasing productivity.
In architainment, the hybrid of entertainment and architecture, designers are installing customized lighting in hotels, restaurants, lounges, and nightclubs, as well as architectural lighting for office buildings, shops, and private residences. These market segments are showing enormous growth. In the future, retrofitting will become increasingly important, as LEDs become the standard light sources for general lighting and gradually supplement or replace conventional light sources.
Outdoor Lighting
Outdoor applications for solid-state lighting include building facades, floodlights, landscaping, public places, parking lots, and street lighting. Use of SSL enables high-quality street lighting with better visibility, natural color rendering, less glare, and high application efficiency. LEDs are also considered promising in this area due to their long service life and reduced maintenance intervals.
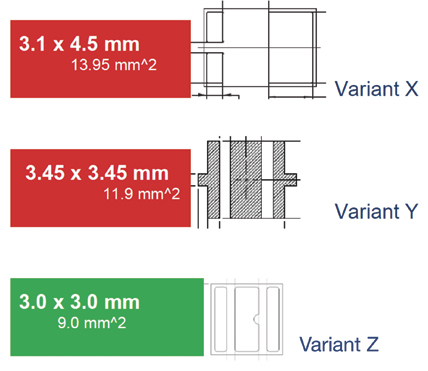
Fig. 3: LED package sizes come in a variety of compact sizes; those above from three LED manufacturers range from 3 x 3 mm to 3.1 x 4.5 mm.
Through custom optics, light can be targeted to a specific area or position, providing more uniform coverage, eliminating hot spots and spill-light, and reducing up-light, which is a major contributor to light pollution. LEDs with an integrated lens can be employed that eliminate the need for secondary optics, making the design process easier, and the silicone lens materials used can also ensure long lifetime and reliable performance [Figs. 4(a) and 4(b)].
LEDs for SSL outdoor lighting are fully dimmable so the light output can be adapted to the light level of the surrounding environment, saving electricity and reducing light pollution. The use of ambient-light sensors can enable streetlights to adjust light levels based on how dark it is. Ambient-light sensors (as shown in Fig. 5) are photodetectors that are designed to perceive brightness in the same way the human eye does and are proving to be a most effective solution compared to standard a-Si wherever the settings of a lamp need to be adjusted to ambient-light conditions as they would be perceived by humans. In addition, proximity sensing can enable streetlights to change intensity when a person or vehicle is detected. Proximity sensing can be achieved by using infrared emitters and detectors.
Conclusion
LED-based lighting continues to gain momentum against conventional lighting. LEDs are already well-established in areas such as architainment and hospitality as well as digital signage. Now, they are rapidly expanding into general lighting in dozens of mainstream applications (such as street, shop, office, and home) where they provide superior luminance, more genuine light, greater energy efficiency, color control, minimal heat, and longer life, with fewer environmental issues. And as the price/performance ratio of LEDs continues to improve, their higher upfront cost is becoming less and less of an obstacle to increased market penetration.
References
1Source: U.S. Dept. of Energy MYPP (Multi-Year Program Plan) and survey of commercial device prices.
2http://www.osram-os.com/osram_os/EN/ About_Us/We_shape_the_future_of_light/ Our_obligation/LED_life-cycle_assessment/ LED_Benefits/index.html ).
3U. S. Department of Energy.
4Understanding IES LM-79 & IES LM-80, by Eric Richman, Pacific Northwest National Laboratory, U. S. Department of Energy.
5U. S. Department of Energy. •
(a)
(b)
Fig. 4: Light can be targeted through custom optics, such as employed in (a) OSRAM's Golden Dragon Oval Plus used in (b) the street scene. Its oval radiation pattern delivers directed light, putting the light where it is needed on the road surface for homogeneous illumination.
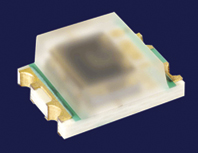
Fig. 5: Spectral sensitivity of a standard Si detector and an ambient-light sensor (SFH 5711 inset) are compared to the human eye (V-lambda).
Chris Eichelberger is an Application Engineer, Solid-State-Lighting/LED Products, for OSRAM Opto Semiconductors. He can be reached at Christopher.Eichelberger@osram-os.com.