Holographic Laser Projection Technology
Conventional lamp-based imaging projection technology is unable to simultaneously satisfy the demands from consumer-electronics manufacturers for projectors that are small, low in cost, con-sume little power, and offer a robust implementation, all while providing high-quality images. A number of LED- or laser-based microprojector technologies are now being developed to address these issues. Here, one manufacturer details its approach – a unique holographic laser projection technology that offers advantages over imaging and scanned-beam display technologies.
by Edward Buckley
THE CONVENTIONAL imaging projector is a near-ubiquitous device in today's offices and is also commonly found in cinemas and homes. Despite the capabilities of such projection systems, technical limitations in terms of miniaturization and power efficiency currently prevent the widespread adoption of projection subsystems into consumer-electronics (CE) and automotive applications.
The need for small, power-efficient projectors in the CE space is aptly demonstrated by the advent of mobile TV. It is clear that the high-resolution content available is incompatible with a typical cell-phone display of 2.5-in. diagonal and, as consumers and manufacturers alike have long since realized, such a display format is inconvenient for sharing content with multiple viewers. These restrictions could be solved by employing a battery-powered projection accessory or embedded projection device to display the content.
Applications such as reconfigurable instrument clusters and head-up displays (HUDs) in the automotive sector are also creating demand for miniature projection systems. Whilst there are clear benefits in using projectors for both applications, similar barriers to integration also apply because – as in the CE space – conventional projection systems are generally unsuitable for integration. For example, in addition to the high brightness and contrast ratios required for HUD applications1 and extremely tight space constraints for rear-projection instrument cluster displays,2 the entire projection subsystem must be robust, fault tolerant, and optically efficient while maintaining wide operating and storage temperature ranges.
As a result, a number of LED- or laser-based microprojector technologies are now being developed to address these issues and to overcome the fact that conventional lamp-based imaging projection technology is unable to simultaneously satisfy the key requirements of small physical size, low cost, low power consumption, and a robust implementation. In addition, it is clear from discussions with automotive and CE customers that there are several additional, and perhaps more restrictive, requirements that also must be fulfilled for the commercialization and acceptance of such miniature projection displays:
• High resolution
• High brightness
• Low speckle
• Eye safe
• Large depth of focus and wide projection angle.
Light Blue Optics (LBO) has been developing a unique holographic laser projection technology since 2004 that, unlike imaging and scanned-beam display technologies, has the unique ability to simultaneously fulfill the key OEM requirements outlined above.
Holographic Laser Projection
LBO's technology represents a revolutionary approach to the projection and display of information. Unlike other commercially available projection technologies, this projection engine exploits the physical process of two-dimensional diffraction to form video images.
A typical imaging projection system works by displaying a desired image Fxy on a micro-display, which is usually sequentially illuminated by red, green, and blue light to form color. In this case, the microdisplay simply acts to selectively block (or amplitude modulate) the incident light; after passing through magnification optics, the projected image Fxy appears. Conversely, holographic laser projection forms the image Fxy by illuminating a diffraction (or hologram) patternhuv by laser light with a wavelength of λ. If the hologram pattern is represented by a display element with pixel size Δ, then the image Fxy formed in the focal plane of the lens is related to the pixellated hologram pattern huv by the discrete Fourier transform F[·] and is written as
Fxy= F[huv] (1)
as shown in Fig. 1.
The key task in a holographic projection system is to compute the hologram huv; a reasonable first guess might be to calculate the inverse Fourier transform of the image Fxy to obtain the desired result. However, the result of this calculation would be fully complex, and there is no liquid-crystal (LC) material in existence that can independently and continuously modulate both amplitude Auv and phase φuv where huv = Auvexpjφuv. Even if such a material became available, the result contains amplitude components that would absorb incident light and reduce system efficiency. A much better approach is to restrict the hologram huv to a set of phase-only values φuv such that huv = expjφuv. As a result, when the hologram patterns are displayed on a phase-modulating microdisplay and subsequently illuminated, no light is blocked.
LBO's system uses a custom-manufactured ferroelectric-liquid-crystal–on–silicon (LCoS) microdisplay from Displaytech, Inc., to display the hologram patterns, which requires that the hologram phase φuv is quantized to a set of binary values. This procedure inevitably introduces quantization noise into the resultant image Fxy, which must be mitigated in order to maintain high image quality. Thus, the microdisplay is used to display N independent holograms per video frame within a temporal bandwidth of the eye of 40 msec, each of which produces a sub-frame Fxy exhibiting statistically independent quantization noise.3 If the intensity of the ith displayed image is I = ׀Fxy׀2, then the time-averaged percept over N sub-frames is
(2)
which is noise-free, as illustrated in Fig. 2.
Uniquely, the key to this holographic laser-projection technology lies not in the optical design but in the algorithms used to calculate the phase hologram huv from the desired image Fxy. LBO has developed and patented proprietary algorithms for the purposes of calculating N sets of holograms per video frame, both efficiently and in real time, as first demonstrated in 2004.4 Crucially, such algorithms can be efficiently implemented in a custom silicon chip.
A practical realization of a holographic laser projector is rather simple and is shown in the schematic of Fig. 3. A desired image is converted into sets of holograms by LBO's proprietary algorithms and displayed on a phase-modulating microdisplay that is time-sequentially illuminated by red, green, and blue laser light, respectively. The subsequent diffraction pattern passes through a lens pair L1 and L2, which is chosen to provide an ultra-wide projection angle in excess of 100°. As a result of the phase-modulating microdisplay, the incident light is steered into the desired image pixels – without blocking – and, due to Fourier optics, the image remains in focus at all distances from the lens L2.
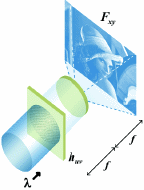
Fig. 1: The relationship between hologram huv and image Fxy present at the back focal plane of a lens of focal length f, when illuminated by coherent monochromatic light of wavelength λ.

Hologram
|
Subframe
|
Frame
|
huv = exp jφuv
|
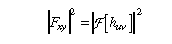
|
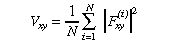 |
Fig. 2: The relationship between hologram huv, sub-frame Fxy, and frame Vxy in LBO's holographic projection technology.
Advantages of Holographic Laser Projection
Low Speckle Contrast: One of the huge advantages of LBO's technology is the ability to substantially reduce laser speckle, a phenomenon that makes the image "sparkle" due to scattering of coherent light from an optically rough projection surface and subsequent interference at the retina. The ability to reduce speckle is important because, not only do users find the artifact very unpleasant, it also severely impacts the perceived image quality and effective resolution.
Although there have been several demon-strations of speckle reduction in the literature,5-10 thus far only LBO has demonstrated the possibility for speckle reduction within the projection optics of a miniature laser projector.
As has been shown previously,3 several methods can be combined in the LBO projector in order to reduce speckle. The first results from the method of image generation and display used in the LBO system. Since N phase-independent sub-frames per video frame are shown within the eye's integration period, then the eye acts to add N independent speckle patterns on an intensity basis, and the contrast of the low-frequency components of the speckle in the field Vxy falls as N1/2. Evidently, some speckle reduc-tion is inherent in LBO's multiple holograms per video frame approach to image display.
Additional methods can be combined to further reduce the speckle contrast because N cannot be increased indefinitely. Several methods11-13 have been demonstrated to remove speckle, but each require that the operation is performed multiple times per laser dwell period. This is straightforward in LBO's system because the laser modulation frequency is low and all pixels are formed simultaneously. In addition, the intermediate image plane formed between L1 and L2 in Fig. 3 makes it simple to embed a speckle-reduction mechanism in the projector optics. The high laser modulation frequency and lack of an image plane make time-varying speckle reduction techniques difficult to implement in a scanned-beam system, as experts in the field have noted.14 These restrictions dictate that speckle in a scanned-beam projector can only be acceptably suppressed by using potentially costly custom diffusing screens, thereby limiting the utility of such systems in CE applications.
A demonstration of the efficacy of LBO's combined speckle reduction techniques is shown in Fig. 4; note that laser speckle is substantially reduced in the projected image, without significant loss of focal depth or resolution.
High Brightness and Efficiency: It has previously been shown15 that, due to the phase-modulating approach to image formation, a holographic display can project significantly brighter images than imaging and scanned-beam systems when displaying video and photo content. In addition, because the image pixels are formed using an expanded beam which has an extremely wide projection angle, it is possible to make a holographic laser projection system much brighter than a scanned-beam display for the equivalent laser safety classification.
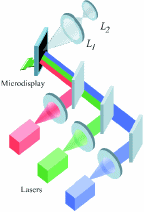
Fig. 3: A schematic diagram of LBO's projection technology.
(a)
(b)
(c)
(d)
Fig. 4: A sample image from LBO's projector (a) without speckle removal and (b) using a combination of speckle reduction techniques. The close-ups (c) and (d) clearly show substantial speckle reduction.
The same combination of laser and phase-modulating hologram provides a highly power-efficient method of projection since, unlike imaging displays, no light is blocked inthe system. Furthermore, the phase-modulating nature of the microdisplay means that it is not necessary to continuously illuminate the micro-display; the lasers are modulated in accordance with the frame brightness, thereby only utiliz-ing the power required to illuminate "on" pixels in the same way as a scanned-beam system.
Although LBO's projection technology carries the overhead of hologram computation, the overall system efficiency is expected to be comparable to that of scanned-beam systems. This is principally due to the low frequency at which the lasers are modulated in LBO's system; since gray scale is formed by the hologram and because the phase-modulating microdisplay directs a fixed proportion of light onto the image, it is only necessary to modulate the laser sources with respect to average video-frame brightness. This necessitates a laser modulation frequency on the order of 1 kHz, allowing efficient digital modulation schemes to be used for each color.
This is significantly more efficient than the method typically employed by scanned-beam projectors, where the pixel-by-pixel method of image formation requires the lasers to be switched at high currents and frequencies of tens of MHz using complex and power-hungry circuitry. Furthermore, limitations in green-laser switching speed also necessitate that the laser is inefficiently analog modulated above and below threshold to render gray scale.
High Resolution, High Image Quality, and Wide Color Gamut: In imaging systems, it is difficult to achieve high resolutions while maintaining an acceptable form factor because field breakdown, diffractive effects, and étendue-matching considerations set the minimum pixel size of the microdisplay.16 The resolution of scanned-beam systems, on the other hand, is principally limited by the achievable laser modulation frequency.
In LBO's system, the resolution of the image is decoupled from that of the micro-display and is controlled largely by the hologram computation algorithm; this allows the resolution to be fully variable up to a maximum of WVGA using just a 7 mm ´ 7 mm active-area microdisplay. Furthermore, the holographic system does not have a 1:1 correspondence between microdisplay pixels and projected image pixels as imaging systems do; in fact, each pixel on the microdisplay contributes to every pixel in the image, making the system tolerant to microdisplay defects.
It is generally accepted that microdisplay-based technologies, whether LCoS or DLP-based, have the capability to offer higher image qualities than scanned-beam systems.17 Scanned-beam systems tend to suffer from poor image quality due to the unacceptably high speckle contrast ratios,18,19 scanning artifacts,20 and image distortion, making the use of such a technology unfeasible in applications where high image quality is required.
It is well known that laser sources can pro-vide images with extremely wide color gamuts, due to their narrow spectral bandwidth; the Helmoltz-Kohlrausch effect can also increase perceived brightness due to the psychophysicaleffects of highly saturated primaries. RGB LED systems exhibit an acceptable color space for CE applications, although systems that use white LEDs have significantly reduced gamuts due to the strong absorption of the microdisplay color filters at red wavelengths.
Conclusion
LBO's holographic laser projection technology represents a revolutionary approach to the projection and display of information, exploiting the physical process of two-dimensional diffraction to form video images. Such an approach simultaneously provides many compelling benefits compared to competing LED- and laser-based miniature projection systems, and the ability of this technology to satisfy the stringent requirements outlined by CE and automotive customers will allow LBO to bring this truly disruptive display technology to market in 2009.
References
1M. Moell, "Color HUD for Automotive Applications," Technical Report, Troy, MI.
2E. Buckley, "Color holographic laser projection technology for heads-up and instrument cluster displays," Proc. 14th Annual Vehicle Displays Symposium (2007).
3E. Buckley, "Holographic laser projection technology," SID Symposium Digest 39, 1074–1078 (2008).
4A. J. Cable, E. Buckley, P. Mash, N. A. Lawrence, T. D. Wilkinson, and W. A. Crossland, "Real-time binary hologram generation for high-quality video projection applications," SID Symposium Digest 35, 1431–1433 (2004).
5D. Gabor, "Laser speckle and its elimination," IBM J. Res. Dev., 509–514 (1970).
6L. Wang, T. Tschudi, T. Halldorsson, and P. R. Petursson, "Speckle reduction in laser projection systems using diffractive optical elements," Appl. Opt. 37, 1770–1775 (1998).
7J. I. Trisnadi, "Speckle contrast reduction in laser projection displays," Technical Report (2003).
8J. I. Trisnadi, "Hadamard speckle contrast reduction," Opt. Lett. 29, No. 1, 11–13 (2004).
9A. Mooradian, S. Antikichev, B. Cantos, G. Carey, M. Jansen, S. Hallstein, W. Hitchens, D. Lee, J. M. Pelaprat, R. Nabiev, G. Niven, A. Shchegrov, A. Umbrasas, and J. Watson, "High power extended vertical cavity surface emitting diode lasers and arrays and their applications," Micro-Optics Conference (Tokyo) (2005).
10B. Dingel, S. Kawata, and S. Minami, "Speckle reduction with virtual incoherent laser illumination using a modified fiber array," Optik 94, 132–136 (1993).
11S. Lowenthal and D. Joyeux, "Speckle removal by a slowly moving diffuser associated with a motionless diffuser," J. Opt. Soc. Am. 61, 847–851 (1971).
12Y. Imai and Y. Ohtsuka, "Laser speckle reduction by ultrasonic modulation," Opt. Commun. 27, 18–22 (1978).
13L. Wang, T. Tschudi, T. Halldorsson, and P. Petursson, "Speckle reduction in laser projections with ultrasonic waves," Opt. Eng. 39, No. 6, 1659–1664 (2000).
14J. W. Goodman, Speckle Phenomena in Optics: Theory and Applications (2008), p. 223.
15E. Buckley, A. Corbett, P. Surman, and I. Sexton, "Multi-viewer autostereoscopic display with dynamically addressable holographic backlight," SID Symposium Digest 39, 340–344 (2008).
16E. Buckley, A. Cable, T. Wilkinson, and N. Lawrence, "Viewing angle enhancement for two- and three-dimensional holographic displays using random super resolution phase masks," Appl. Opt. 45, No. 28, 7334–7341 (2006).
17Insight Media LLC, "Laser Projection Systems 2007.
18M. Handschy, "Moves toward mobile projectors raise issue of panel choice," Display Devices, 6–8 (2007).
19M. Schmitt and U. Steegmuller, "Green laser meets mobile projection requirements," Optics and Laser Europe, 17–19 (2008).
20Insight Media LLC, "Large Display Report," pp. 71–72 (June 2008). •
Edward Buckley is VP of Business Development at Light Blue Optics, 4775 Centennial Blvd., Suite 103, Colorado Springs, CO 80919, USA; 719/623-1208 , e-mail: edward@lightblueoptics.com.