Diagnostic Systems for Pregnancy Healthcare through Telemedicine Networking
Diagnostic Systems for Pregnancy Healthcare through Telemedicine Networking
Portable sensors and wireless networks are creating new applications in the field of pregnancy healthcare and fetal-wellness monitoring. Portable devices and smartphones can be used as network coordinators and user interfaces for remote diagnoses from home.
by Giorgio De Pasquale and Angela Lentini
TELEMEDICINE – the remote diagnosis and treatment of patients via telecommunications technology – has been undergoing rapid development, thanks to the spread of small and low-power devices, distributed wireless networks, and portable communication systems. By using telemedicine procedures, it will soon be possible to share medical data through secured protocols between patients and medical providers.
The main benefits of telemedicine include cost savings, in terms of fewer hours worked by doctors and nurses and lower occupancy of hospital beds, and lifestyle enhancement because patients will be able to make fewer trips to healthcare facilities for routine exams.
The healthcare processes supported by telemedicine can be described accordingly:
• Prevention: This service is very important for people affected by high-risk disorders; for example, diabetes and cardiac diseases.
• Diagnosis: It is possible to obtain a diagnosis without leaving home.
• Therapy: This process is probably more far off in the future than the other three listed here; the goal is to automatically adapt the appropriate medical therapy to the patient’s health conditions.
• Rehabilitation: This patient service is applied after hospitalization and can be used in nursing
homes, rehabilitation centers, or the patient’s home. An example might be for the monitoring of mobility recovery after orthopaedic operations.
The most important available tools of telemedicine are
• Telemonitoring: This service allows the monitoring of vital parameters of patients, particularly when they are affected by serious pathologies.
• Teleconsulting: This service occurs between patients and health workers, with video support for communication.
• Telereporting: This service refers to data transmission between two different health workers who need to compare their ideas about a patient’s condition.
• Teleconferencing: This service occurs among a group of health workers who use video conferencing to discuss a patient’s pathology.
Of the above telemedicine services, telemonitoring is the most important and has three main phases: (1) acquisition of clinical data from the patient about his or her condition, (2) sending of patient’s data to medical centers or to data storage for further processing and evaluation, and (3) sending of feedback message to the patient about the received/stored clinical data.
This service is used for monitoring the vital parameters of patients, in particular when they are affected by serious pathologies. Specific data can be measured continuously or at fixed intervals. Once data has been delivered, medical personnel can perform data analysis. A typical structure for such a service, consisting of sensors mounted on patients, a mobile device to capture the information from the sensors, and a healthcare service to receive the data from the device, appears in Fig. 1. This structure is generally known as a wireless biomedical sensors network (WBSN), and it is basically an architecture of sensors (the nodes) that communicate with a gateway central node (the coordinator or mobile device) that
receives the information and then sends it to a healthcare service.1,2 A WBSN, which can be used for different applications, allows patients to move freely, thanks to the small size and light weight of the nodes and the wireless connections among them. Such a system is capable of providing not only greater comfort for patients, but economic advantages for healthcare systems.
Some portable WBSNs for pregnancy diagnosis have been proposed, but the complexity of the measurements requires additional efforts in improving existing systems reliability and accuracy. The device described in this article is at the prototype stage. It demonstrates high portability, ease of use, natural interfacing with portable electronics, and reliable data management.
Many digital devices can be used to receive measured data from the distributed sensors network on the patient’s body, by means of short-range wireless communication. For instance, portable devices such as smartphones and tablets, which are now common in hospital settings as well as homes, can work as network coordinators through simple software applications and Bluetooth/WiFi ports. When the measured values reach a given threshold level, a first-warning message
is sent to the hospital (Fig. 1); the number of warnings and their timing intervals will suggest the next move – a doctor might be advised to call the patient at home, for example, and/or have her come to the hospital.
The most important telemonitoring services are in the area of cardiac pathologies, dialysis, and diabetes. The fundamental physiological parameters detected generally include heart rate, blood pressure, blood-oxygen saturation, movements, and breathing. These services already use biomedical wearable sensors, which have very small dimensions and low power consumption, in clinical settings. They are generally user-friendly, efficient, reliable, and safe. They also allow the monitoring of physiological parameters for long periods using rechargeable batteries. In addition, researchers have recently proposed power generation that would occur directly on the human body by means of electro-mechanical transducers (energy harvesters) based, for instance, on piezoelectric materials.
The small dimensions of sensors suggest the possibility of developing smart clothes with embedded electronic components for the monitoring of vital parameters. Smart clothes would also presumably allow a more transparent and continuous (rather than sporadic, through attached sensors) monitoring of physiological parameters, and thus provide more alerts to emergency services when those parameters exceeded given thresholds. This would not only save lives but bring about a reduction in damage caused by acute events such as heart attacks.
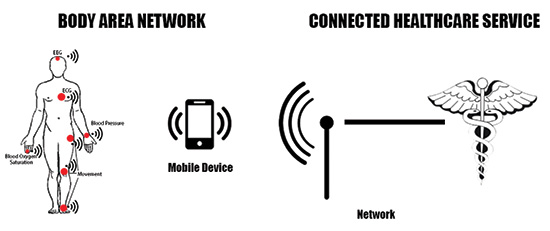
Fig. 1: In this potential structure for telemedicine services, the distributed sensors network sends the measured data to the local network coordinator (represented, for instance, by a smartphone), which is connected to the Internet for sharing data through secured protocols with medical centers.
Fetal-Wellness Project
We believe that the remote diagnostic of fetal heart wellness during pregnancy is an ideal and innovative application of WBSN. In fact, solutions based on wearable sensors and data networking could provide significant benefits for the remote diagnostic of pregnant patients through self-examination at home. At this time, several commercial wearable biomedical sensors for pregnancy monitoring are available.3–8 Portable communication devices (such as mobile phones) have several software applications supporting connections with external sensors as well as the possibility of storing the measured data. However, these simple networks are limited to the sphere of personal use and have no measured medical value. In order to validate this potentially useful application, our research group is working on new types of smart sensor networks for fetal heart-rate detection that are able to provide the sensitivity and sampling frequency typical of professional instruments.9,10
Our research group has worked for several years on self-powered and wearable sensors.11–14 Our current goal is to apply telemedicine to a specific medical field of obstetrics: the fetal heart telemonitoring project briefly described in this article is aimed at developing prototypes for wearable sensors with wireless Internet connection for cardiotocography (CTG) examinations during pregnancy.
The methodology and facilities for pregnancy examinations have changed little over many years, while innovations introduced by telemedicine have the potential to significantly improve the efficiency of hospital practices and to reduce the associated cost and effort on the part of healthcare workers. Some examples of portable sensors for telemedicine have been introduced in the literature. For instance, the cardiotocograph by Korostelev et al.15 connects a pressure sensor and an ultrasonic sensor to portable devices using the Android operating system. A system developed by Boeing et al.16 for pregnancy monitoring detects the fetal heart sound with the principle of the stethoscope, in which the sound waves coming from the patient’s body are captured by a microphone and used to interpret the heartbeat of the fetus. And a paper from C. Tufo from the University of Naples17 presents some algorithms for the post-processing of cardiotocography data.
Our project started with the statistical investigation of a population of 62 patients in a hospital obstetrics unit, where it had been observed that 78% of patients had to travel more than 10 km for pregnancy examinations; and 39% of those traveled more than 30 km. Starting from the 36th week of pregnancy, the exam is repeated once per week for all patients; in the last two weeks of the pregnancy, these exams are required one to three times per week for all patients, and in the case of high-risk pregnancies, a frequency of one or more times a day is also possible depending on the specific pathology of the patient. A total of 96% of the patients interviewed expressed a very positive opinion about a home device that would be able to replace the hospital examination; 2% reported a moderately positive impression; and another 2% did not recognize any immediate benefit from this technology. Clearly, these pregnant patients were extremely positive about the idea of fetal hearth telemonitoring. This survey was conducted by referring to an ideal telemonitoring system that was not in use at the time of the interviews, but these results provide additional motivation regarding the development of the system.
The examination is called “cardiotocography” and is based on the measurement of the fetal heartbeat regularity. It requires two modes of detection: one for the contractions of the patient and another for the heartbeat of the fetus (Fig. 2). The exam takes from 45 to 100 minutes and requires the patient to stay perfectly still. Detection made with the hospital instrumentation is almost completely automatic; however, large and heavy devices must be used and the assistance of specialists (nurses and technicians) is mandatory. One of the main challenges associated with remote cardiotocography is miniaturizing the instrumentation without losing important information. The sensing strategy of portable instruments is different and less accurate than that of instruments operated in hospitals – we are striving to preserve the integrity of the most important data, which can
identify the necessity of further and more accurate analysis. The detection modes are similar to those at home in that the patient cannot move around but the difference is they are completely automatic, do not require specialist’s assistance, and do not require a trip to the hospital.
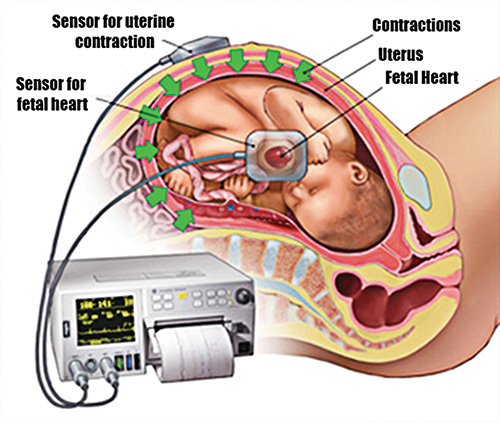
Fig. 2: A traditional system for cardiotocography presently used in hospitals’ obstetric units incorporates a sensor for contractions and a sensor for the fetal heartbeat.
The portable monitoring device being developed by our team also uses two types of sensors: one for fetal heartbeat monitoring and one for uterine-contraction
monitoring. The sensors are wireless and can communicate with traditional portable communication devices (smartphones, tablets, smart watches, etc.). The cardiotocography data are reported on the portable device display as they are measured before being sent via the Internet to the obstetric unit of the hospital for detailed analysis. Finally, the cardiotocography data are stored in the patient database. Remote fetal health monitoring is designed to use secure protocols to share measurements between the patient and the medical provider. If issues are indicated during the routine examination, the patient must then
move to the hospital for full analysis.
The block diagram shown in Fig. 3 shows the workflow of the remote system for the diagnosis of the fetal heart rate. When the sensors are activated, they send data to the portable device, which processes the received information and also verifies the proper positioning of sensors on the body (through the evaluation of signal noise). In case of incorrect positioning, an error message sent to the mobile devices
display informs the user. Otherwise, the data are measured, temporarily stored, and processed. The implemented algorithm is able to detect the occurence of unexpected measurements and to recognize isolated or repeated anomalies. In the first case, the smart device sends the processed data to the hospital obstetric unit. In the second case, a warning message (indicating the possibility of wrong/missed measurement or over-threshold value) is displayed on the portable device before sending the data to the hospital. Then, if alterations in cardiotocography are detected, the emergency medical service is activated for direct assistance
at the patient’s home.
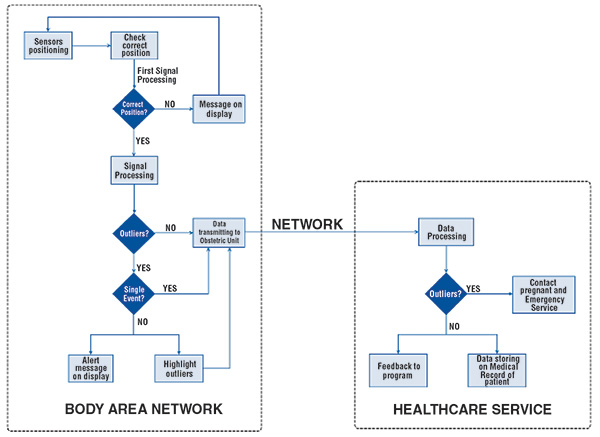
Fig. 3: The proposed system for the monitoring of fetal heart rates incorporates different protocols for abnormalities vs. “normal” results.
The portable telemonitoring system is composed of three main parts: the sensor, the electronics, and the mobile device. The electronic circuit (Fig. 4) includes a microprocessor, a wireless transmission port, and two batteries for the power supply. The overall dimensions of the circuit are 100 × 60 × 30 mm. Figure 5 shows the architecture of the telemonitoring system and Fig. 6, the application interface for smartphone displays.
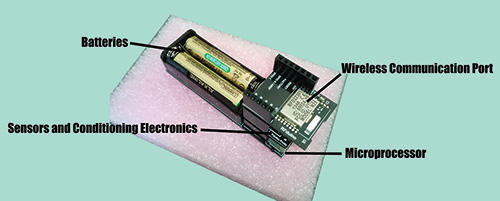
Fig. 4: One node of the distributed sensors network includes the wireless transmission unit (top level), the sensors circuit (middle level), and the microprocessor (low level); two rechargeable batteries provide the power supply.
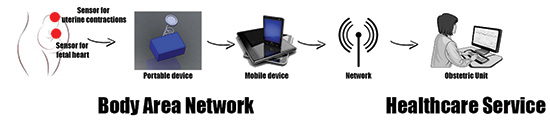
Fig. 5: The structure for the proposed fetal heart-rate monitoring system also incorporates a portable hardware node that would receive the data before sending it on to the mobile device.
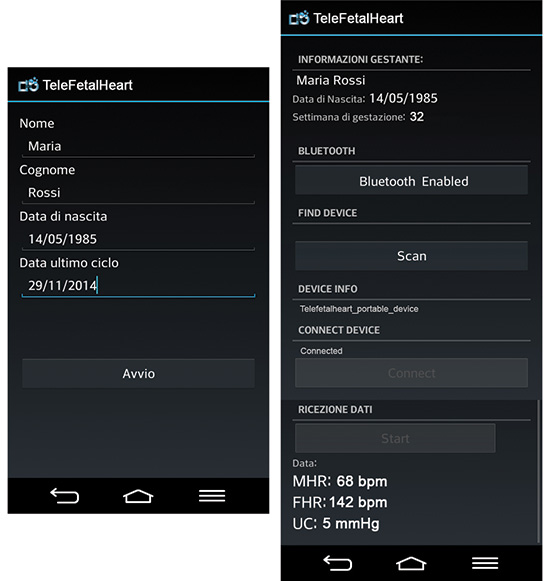
Fig. 6: This graphical interface for the application developed to support the telemonitoring system would appear on smartphone/tablet displays.
Challenges and Possibilities
The rapid evolution of telemedicine and wearable sensors technology is opening up the quality and convenience of care for patients, including and especially pregnant women. Among the main benefits of this progressive development is a reduction in healthcare systems costs. The positive impacts on the lifestyles of patients who would not have to travel long distances to healthcare centers are also of major benefit.
The system described in this article is in the prototype stage; the firmware and circuits have been optimized for operating in controlled conditions. The next phase will address the improvement of system reliability, the cost reduction of components, reduced packaging size, and certification. The system has been tested on the human body by volunteer researchers who measured heart rates and blood pressure. In future applications, particular effort will be given to the user interface
and subsequent reduction of false positive warnings – this depends to a great degree on the way the sensors are set up on the body. The non-specialist use of the device must include very easy learning procedures and clear responses from the system.
There are no particular limitations with regard to the diffusion of the system, except for technical issues associated with the GSM network coverage of geographical areas in question. The telemonitoring strategy exploited by the system developed in this work has many potential applications in medical examinations beyond fetal healthcare. For instance, the remote diagnosis of pathologies related to glycemia, postural recovery after traumas, skin, eyes, throat, etc., can be supported by the same electronic hardware and dedicated sensors and firm-ware.
The development of displays specifically designed for these applications — which might include those of very high resolution and low power — can improve the global performances of the system. We believe the telemedicine market is very promising for the development of the display sector. And, of course, we believe that using currently available remote fetal heart-monitoring technology, telemedicine is a likely alternative for hospital obstetric units worldwide.
References
1P. E. Ross, “Managing care through the air,” IEEE Spectrum 41, No. 12, pp. 26–31 (December 2004).
2D. Malan, T. Fulford-Jones, M. Welsh, and S. Moulton, “CodeBlue: An Ad Hoc Sensor Network Infrastructure for Emergency Medical Care” (2004).
3E. Jovanov, A. O’Donnell Lords, D. Raskovic, P. G. Cox., R. Adhami, and F. Andrasik, “Stress Monitoring Using a Distributed Wireless Intelligent Sensor System,” IEEE Eng. Med. Biol. Mag. 22, Issue 3, 49–55 (2003).
4T. Martin, E. Jovanov, and D. Raskovic, “Issues in Wearable Computing for Medical Monitoring Applications: A Case Study
of a Wearable ECG Monitoring Device,” Proc. 4th Intl. Symposium on Wearable Computers, 43–49 (2000).
5M. Milenkovic, E. Jovanov, and J. Chapman, “An Accelerometer-Based Physical Rehabilitation System,” Proc. 34th Southeastern Symposium on System Theory, 57–60 (2002).
6R. Isais, K. Nguyen, G. Perez, R. Rubio, and H. Nazeran, “A low-cost microcontroller-based wireless ECG-blood pressure telemonitor for home care,” Engineering in Medicine and Biology Society, Proceedings of the 25th Annual International Conference of the IEEE, 3157–3160 (2003).
7P. Bonato, P. J. Mork, D. M. Sherrill, and R. H. Westgaard, “Data mining of motor patterns recorded with wearable technology,” IEEE Engineering in Medicine and Biology Magazine 22, No. 3, 110–119 (May 2003).
8A.Caduff, “First human experiments with a novel non-invasive, nonoptical continuous glucose monitoring system,” Biosensors and Bioelectronics 19, No. 3, 209–217 (November 2003).
9R. Paradiso, “Wearable Health Care System for Vital Signs Monitoring” Proc. 4th Ann. IEEE Conf. on Information Technology Applications in Biomedicine, 283–286 (2003).
10D. De Rossi, F. Carpi, F. Lorussi, A. Mazzoldi, R. Paradiso, E. P. Scilingo, and A. Tognetti, “Electroactive fabrics and wearable biomonitoring devices,” AUTEX Research Journal 3, No. 4 (December 2003).
11G. De Pasquale and A. Somà, “Energy harvesting from human motion with piezo fibers for the body monitoring by
MEMS sensors,” Proc. Symposium on Design, Test, Integration and Packaging of MEMS/MOEMS, DTIP 2013, Barcelona, Spain, 79–84 (16–18 April 2013).
12G. De Pasquale, Biomechanical Energy Harvesting, “Design, testing and future trends for healthcare and human–machine interfaces,” Innovative Materials and Systems for Energy Harvesting Applications, edited by L. Mescia, O. Losito, and F. Prudenzano, Chapter 11, IGI Global
(2015).
13G. De Pasquale, S. G. Kim, and D. De Pasquale, “GoldFinger: wireless wearable human–machine interface powered by biomechanical energy harvesting with dedicated software,” IEEE/ASME Transactions on Mechatronics (in press).
14G. De Pasquale, “Artificial human joint for the characterization of piezoelectric transducers in
self-powered telemedicine,” Applications, Meccanica (in press).
15M. Korostelev, L. Bai, J. Wu, C. C. Tan, and D. Mastrogiannis, “Body Sensor Networks in Fetal Monitoring with NFC Enabled Android Devices,” Proceedings of the 7th International Conference on Body Area Networks, 9–12 (2012).
16J. Boeing, T. Gatlin, E. Hendler, and E. Hyde, Fetal Heart Rate Monitor for Resource-Limited Settings (University of Michigan, 2012).
17C. Tufo, “Sviluppo e confronto di algoritmi di filtraggio del segnale fonocardiografico addominale per l’estrazione della frequenza cardiaca fetale,” Tesi di Laurea Specialistica, Università degli Studi di Napoli – Federico II (2011). •
Giorgio De Pasquale and Angela Lentini are with the Department of Mechanical and Aerospace Engineering at the Politecnico di Torino in Italy. De Pasquale can be reached at giorgio.depasquale@polito.it.