Reflection Performance in Curved OLED TVs
Reflection Performance in Curved OLED TVs
One of the creators of the IDMS (Information Display Measurements Standard) takes the measure of the latest large, curved OLED TVs. In this second article in a series, he looks at reflection performance and makes a discovery that may necessitate a revision to the reflection measurement procedure in the IDMS standard.
by Edward F. Kelley
BECAUSE televisions are usually viewed in some sort of ambient condition, it makes sense to see how well they handle ambient illumination and the surround. In this article, we compare the reflection performance of two recently released curved organic light-emitting-diode (OLED) television (TV) displays: an LG 55EA9800 and a Samsung KN55S9CAFXZA. Both are 55-in. OLED [active-matrix OLED (AMOLED)] units. As discussed in the first article in this series, “Considering Color Performance in Curved OLED TVs,” in the November/December 2013 issue, we say “usually viewed” because there are situations where the ambient influence from the surround is virtually nonexistent, as with a serious gamer in a very dark room. In one way or another, most of us have to contend with rooms with ambient light.
In keeping with the testing methodology from the first article, only one display from each manufacturer was measured, so a statistical sampling was not provided.1,2 Also, keep in mind that manufacturing details can change as newer displays are released and their properties can change.
Lastly, during the course of testing, we discovered that a complete reconsideration of the material in Chapter 11 on reflection in the IDMS document and especially the section on ambient contrast is almost certainly required. Read on.
Quick Visual Inspection with Point Source
One of the best ways to visually inspect the reflection properties of any display is to use a point source. Figure 1 shows a quasi-Lambertian light-emitting diode (LED) in front of and between the two OLED displays. (A flashlight with a bare bulb will work similarly.) Your eye can see much more than this picture can possibly show. The dynamic range of these point-source reflections can be from 105 to 107 (and possibly more), and you can see that range of luminance with your eye – it is essentially a type of bidirectional reflectance distribution function (BRDF) where the source and target remain fixed and the detector observation direction changes.
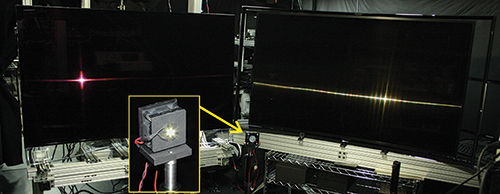
Fig. 1: Point-source reflection is shown with the LG display on the left and the Samsung on the right. The inset shows the front of the LED point source. The displays are in a normal viewing arrangement with their surfaces tilted back a few degrees.
To capture that same dynamic range with photography requires multiple images of varying exposures.3 What we see in these point-source images tells much of the story about the display reflection properties: We see primarily a specular (regular, mirror-like) reflection with matrix scatter in both displays. The LG display is apparently employing an anti-reflection front surface (a magenta hue), and the Samsung display exhibits a very wide matrix-scatter distribution. Neither display has a strong diffusing front surface, so there is essentially no haze component of reflection. It would appear from this photograph that we will see more reflection from the Samsung OLED display than the LG OLED display, in general. For a discussion of the various components of reflection, see the IDMS, Chapter 11, Reflection, and especially the Appendix B17 Reflection Models and Terminology.4
White-Screen Anomaly
Usually, we would worry about reflections from both the white and the black displayed colors. Unfortunately, to obtain reliable reflection measurements with white showing it is necessary that the reflected luminance be measurably greater than the display white. This often requires illumination levels that are not commonly encountered in a normal TV viewing experience. The assumption has always been that linearity and superposition hold so that we can make laboratory measurements of these displays to obtain their reflection parameters and then scale and combine the results in appropriate ways to replicate any ambient environment desired (see IDMS § 11.9 Ambient Contrast).
In this case, we observed an anomalous behavior in the Samsung OLED that we have never seen before. Whereas with liquid-crystal displays (LCDs), we expect to see a small difference in the reflection properties between white and black, such would not be expected in OLED displays. However, for the first time, we found that the Samsung OLED exhibited a darkening of white that was somehow proportional to the illuminance level. This made it impossible to reliably measure the reflection properties with the source illuminance levels we would normally use when attempting to measure the reflection from white.
This development is both problematic and a remarkable result in that superposition does not hold true for such large illuminance conditions on this particular OLED display. This change in white luminance with strong illuminance did not occur for the LG OLED display. However, it is important to note that Fig. 2 shows that for illuminances well below 10 klx, the white level on the Samsung OLED is not as affected. For the purposes of this article, we will assume that the reflectance of white and black are the same for the Samsung OLED display; it is simply unfortunate that we could not verify that assumption directly for low illuminance levels. Thus, we will only report the reflection parameters for black for both of these OLED displays and use those parameters accordingly. The white and black reflection parameters are the same for the LG display within the uncertainty of our measurements.
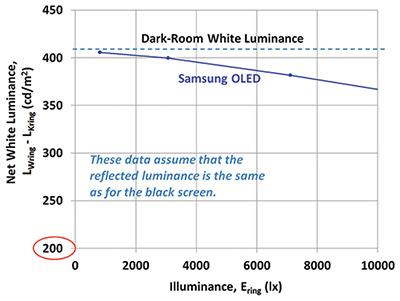
Fig. 2: Assuming that the reflected luminance is the same for black as it is for white, this chart shows what the decrease in white luminance with illuminance for the Samsung OLED display might be.
Reflection Measurement Results
Next, we will examine and compare six reflection properties: diffuse reflectance with specular included, diffuse reflectance with specular excluded, ring-light luminance factor, dual-source luminance factor, specular reflectance for a 15° uniform source, and an in-horizontal-plane BRDF with stationary source and moving detector.5 In all but the BRDF measurements, spectral data are obtained so that spectral-reflectance-factor calculations could be performed; we chose the common photometric reflection parameters for simplicity. In general, the relative expanded uncertainty with a coverage factor of two is 5% for most of these measurements and 10% or more for the BRDF measurements. These displays are tilted back for typical viewing via their frames or mounts; hence, we had to tilt them forward so that their central surfaces were vertical. The reflection for the display turned off and at room temperature might be slightly different than the display being fully warmed up and showing a full black screen. (Any filter material generally has a small change in transmission with temperature.) The difference. if any, is also within the uncertainty of these measurements. However, for all measurements reported here, the displays are warmed up and showing black. A 17-level 25%-screen-size APL pattern with either a white or black center was used until it was established that a full-screen black had the same reflection properties as the APL pattern with a black center.
Table 1 provides the summary of results of this study. Each reflection parameter measurement will be discussed separately below. For all parameters except the specular reflectance and the BRDF maximum, the LG OLED display exhibited less reflection than the Samsung OLED display. When we examine the BRDF profiles as well as the point-source photograph (Fig. 1), we can understand why: The Samsung OLED distributes more of the energy in a wide-angle pattern because of the wide matrix scatter; this results in less energy in the specular direction, whereas the LG OLED display exhibits less matrix scatter and more of the energy remains in the specular direction with less scattered out of the specular direction.
Table 1: These reflection results are based on display black (assumed the same for white).
Reflection Property:
ReflectionParameter:
/
Display |
Diffuse Reflectance |
Luminance Factors |
Specular Reflectance |
BRDF |
Specular Included ρdi/10 |
Specular Excluded ρde/10* |
βring |
βdual |
ζ |
Maximum (sr-1) |
LG OLED |
0.0194 |
0.00113 |
0.00055 |
0.00128 |
0.0224 |
161 |
Samsung OLED |
0.0450 |
0.0282 |
0.0128 |
0.309 |
0.0218 |
127 |
* Note: Because of the presence of matrix scatter, these values of the diffuse reflectance with specular excluded may be very sensitive to apparatus geometry
(size of sphere and size of specular port).
Recall that the term “diffuse” refers to light scattered out of the specular (or regular, mirror-like) direction. In making diffuse-reflectance measurements (using an integrating sphere to provide a uniform hemispherical illumination), we can include the specular component or exclude it via a second hole to not measure the light from the specular direction. Thus, ρdi/10 refers to the diffuse reflectance with specular included measured at 10° from the normal of the sample material, and ρde/10 refers to the diffuse reflectance with specular excluded measured at 10°. For such hemispherically uniform illumination, the diffuse reflectance is given by ρ = πL/E, where L is the net reflected luminance and E is the illuminance. The luminance factor β = πL/E has the same mathematical form, but the net reflected luminance is not from a uniform hemispherical source of illumination but from either a ring light or dual isolated sources in our case. The specular reflectance ζ = L/Ls is the ratio of the net reflected luminance to the source luminance for a discrete source having a subtense of 15°. (This is not strictly the specular reflectance as would be obtained from a high-resolution BRDF measurement, but it is an approximation to the true specular reflectance that is much easier to measure and useful whenever there is a strong specular component. (See IDMS Chapter 11 and § B17 for a full discussion of these reflection components.)
To provide an example of how these reflection parameters might be used in an ambient contrast calculation, we show in Table 2 an example of a TV viewing room as specified in IDMS § 11.9 Ambient Contrast with a hemispherical uniform surround illuminance of 60 lx and another 40 lx possible from isolated lamps in the room. The ambient contrast is the ratio of the white luminance with the reflected luminance added divided by the black luminance (zero for these OLEDs) with the reflected luminance added in. We employed Eq. (1) of IDMS § 11.9 to calculate the ambient contrast. In the last three columns we compare the ambient contrast (1) CA with both the uniform hemispherical surround and the two sources placed at ±30° to maximally interfere with the TV picture, (2) CA′with a hemispherical surround and the ring light to simulate several widely placed sources around the room and ceiling at 45° locations, and (3) CA″without any isolated or directed sources and just the diffuse uniform hemispherical surround. Even with the mild illumination for the TV viewing room, the contrasts are reduced considerably from what is seen in the darkroom. The nice thing about this kind of ambient-contrast calculation in IDMS § 11.9 is that we can change the values as we wish to simulate the performance in other surrounds – such as for a very dark home theater – and recalculate the result. For example, if we only had a hemispherical uniform surround illuminance of 10 lx and no isolated sources, we would have an ambient contrast (third column) CA″of 6460 and 2850, respectively, for the LG and Samsung OLED displays.
Table 2: Example of an ambient-contrast-calculation result.
|
Illuminance Configurations |
Darkroom Luminances (cd/m2) |
Ambient Contrasts |
Both** Ehemi + Edual |
Both Ehemi + Ering |
Only Hemi.Edir = 0 |
|
Ehemi (lx) |
Edir = Edual (lx) |
Edir = Ering (lx) |
|
60 |
40 |
40 |
|
ρdi/10 Ehemi /π (cd/m2) |
βdual Edir/π* (cd/m2) |
βring Edir/π (cd/m2) |
LW |
LK |
CA |
CA′ |
CA″ |
LG OLED |
0.371 |
0.016 |
0.007 |
399 |
0 |
1033 |
1058 |
1078 |
Samsung OLED |
0.859 |
3.93 |
0.163 |
408 |
0 |
86 |
400 |
476 |
* We will assume that there are two such lamps in the ±30° arrangement used to measure the luminance factor for the dual-source configuration.
** Such lamps would also have to be arranged so that their matrix scatter would interfere with the viewed picture area on the TV.
Diffuse Reflectance with Specular Included
The IDMS contains § 11.2 Hemispherical Reflection, Specular Included, and we use here § 11.2.2 Sampling-Sphere Implementation. Figure 3 shows the arrangement for both the sampling-sphere measurement of diffuse reflectance with specular included and excluded.
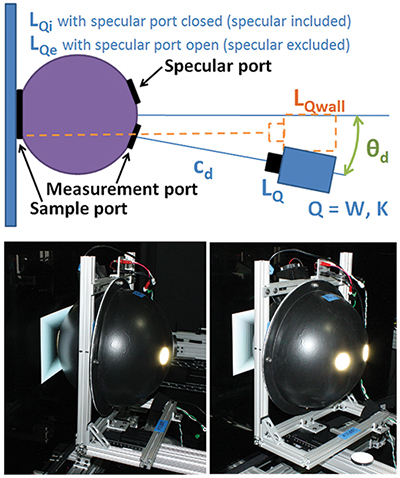
Fig. 3: A sampling sphere was used to measure the diffuse reflectances for both specular-included and specular-excluded configurations. Only Q = K, black, measurements are reported in this article.
Ultimately, we only measured and report here the black reflectances. The white light-emitting-diode (LED) illuminance at the sample port was between 78 and 24 klx (depending upon the LED current setting) based upon wall measurements that were calibrated to have a reflectance of 0.98 according to the procedure in the IDMS § 11.1.3.1 Integrating-Sphere Sources for Uniform-Diffuse Surrounds under item #4 Wall Luminance (Sample Sphere). The spectroradiometer (1° aperture, 4-nm bandwidth) was placed back approximately 1 m from the measurement port of the sampling sphere to avoid any stray light from the wall during sample-port measurements. The angle of the measurement port from the normal of the sample port and TV is 10°. The spectroradiometer can swing sideways to measure either through the
measurement port at the center of the sample port or at the wall next to the sample port. The diffuse reflectances with specular included for the LG and Samsung OLED displays were 0.0194 and 0.0450, respectively.
Diffuse Reflectance with Specular Excluded
Here, we use the procedure outlined in § 11.3 Hemispherical Reflection Specular Excluded and 11.3.2 Sampling-Sphere Implementation (Specular Excluded). With the specular port removed, there is between 72 and 22 klx falling upon the sample port. The measurements proceed as with the specular-included configuration, except
that the spectroradiometer must be carefully positioned so that the virtual image of the specular port is in the center of the sample port and measurement port and so that the measurement is made at the center of the virtual image of the specular port. The display surface at the sample port is kept in focus. The detector distance of 1 m from the measurement port assures no interference from the out-of-focus vignettes of the measurement and specular ports. It is important to note that unless the display reflection is purely specular and/or Lambertian in nature, the size and distance of the specular port may change the measurement result whenever matrix scatter or haze is present in the reflection components. Thus, the specular-excluded measurement results may not be reproducible in other laboratories. The rest of the reflection parameters we measured should be reproducible in other laboratories. The diffuse reflectances with specular excluded for the LG and Samsung OLED displays were 0.00113 and 0.0282, respectively.
Ring-Light Illumination
In the IDMS 11.5 Ring-Light Reflection, suggestions are made regarding the geometry of the apparatus. The ring light used in our tests had a diameter of 197.1 mm, and the requirement for a 45° illumination direction called for it to be placed a distance of 98.6 mm away from the center of the screen. The radial width of the ring of light was 0.44 mm, which gave it a subtense of 0.1°, well under the required <0.5°. At full brightness, the illuminance at screen center was approximately 48 klx from a tungsten-halogen bulb with an IR cutoff filter. Figure 4 shows the steps taken to make ring-light measurements: (a) The ring light was carefully positioned at the proper distance using a special device to gently touch the display without damage. (b) A thin white target whose reflectance was previously calibrated for 45° illumination was placed at the center and its luminance measured to provide the illuminance from the ring light. (c) The measurement of the center region was made with the spectroradiometer placed at a detector distance of approximately 180 cm. (See IDMS 11.1.3.2 Discrete or Directed Uniform Sources and Ring Lights for a suggested method to determine illuminance from a ring light when the display cannot be moved.) Figure 5 illustrates the geometry and shows the view from the detector of both OLED screens under ring-light illumination. Because the LG OLED display has a relatively confined matrix scatter, the measured region is dark. Conversely, the Samsung OLED has a matrix scatter that is widely distributed as manifested by the patterned reflection of the ring light. The ring-light luminance factors for the LG and Samsung OLED displays were 0.00055 and 0.0128, respectively.
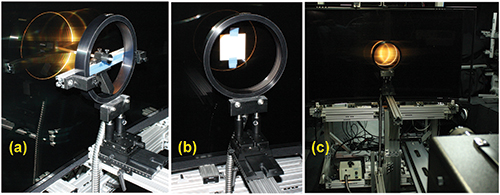
Fig. 4: From left to right are the steps used to make a ring-light reflection measurement.
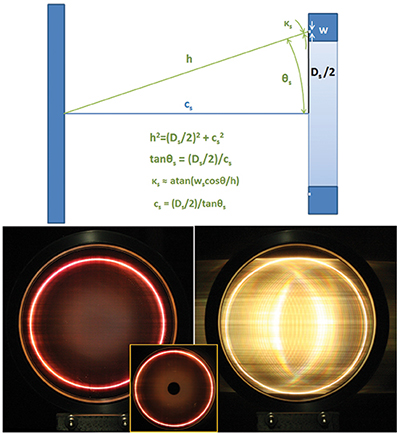
Fig. 5: Ring-light geometry and views from the detector position appear with the LG OLED on the left and the Samsung OLED on the right. The inset picture shows the view through the spectroradiometer eyepiece.
Dual-Source Reflection
Here, we followed the recommendations of IDMS § 11.7.2 Dual-Large Source Reflection, which specify that the sources be placed at distances of 500 mm or more, subtend 15°, and are placed at ±30° on each side of the normal. The detector was positioned to view down the normal of the display (see Fig. 6). We were using box sources with 150-mm-diameter exit ports and tungsten-halogen lamps running at 2856 K. The sources exhibited a non-uniformity of less than 0.25 % (see IDMS 11.1.3.2 Discrete or Directed Uniform Sources and Ring Lights for a suggested uniformity diagnostic). The procedure was similar to that of the ring-light measurement: We obtained the correct distances needed, placed a thin white target on the display surface that had been calibrated for this 30° angle illumination, then measured the reflected luminance with a black screen. The resulting illuminance was 995 lx. Figure 7 shows the appearance of the reflection for both displays from the direction of the normal. The strong matrix scatter of the Samsung OLED results in a rather bright smearing of the sources, but also note the oscillations along the horizontal direction in that reflected light. This oscillation in the luminance increases the uncertainty in the measured reflected luminance. We tried to obtain a reasonable average in the vicinity of center for the Samsung OLED. The dual-source luminance factors for the LG and Samsung OLED displays were 0.00128 and 0.309, respectively. This rather large value for the Samsung OLED display arises from the wide matrix scatter.
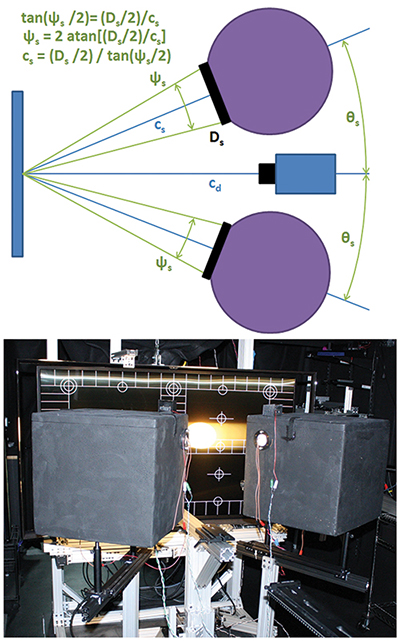
Fig. 6: Dual sources placed at ±30° have subtenses of 15°. The alignment pattern is shown.
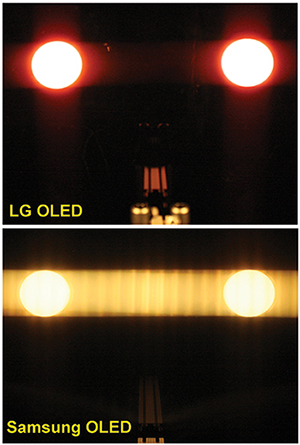
Fig. 7: Here, the reflection from the dual sources is viewed from the normal.
Large-Source Specular Reflection
The IDMS § 11.7.3 Large-Source Specular Reflection requires that the source and detector be placed ±15° on each side of the normal. We used ±30° for ease of apparatus configuration. The source had a subtense of 15° and was identical to the source used on the right side of the dual-source
measurement (see Fig. 8). This type of measurement in the specular direction includes not only the true specular component, but also any haze or matrix scatter in the vicinity of the true specular component. As such, it is not a true measurement of the specular component of reflection whenever non-trivial haze or matrix scatter is present. A high-resolution (approximately 0.2°) BRDF apparatus can resolve the specular component from the other components of reflection, but in this large-source measurement they are lumped together. Accordingly, the symbol ζ is used instead of ρr for this type of specular measurement. The lumped specular reflectance for the LG OLED and Samsung OLED displays were 0.0224 and 0.0218, respectively. To keep this in perspective, a single untreated glass surface specular reflectance is approximately 0.045; so these displays are doing quite well by comparison.
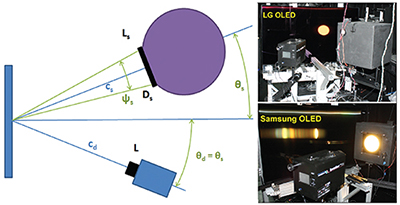
Fig. 8: These images show large-uniform-source reflection measurement in the specular direction. In the picture on the bottom right, the source and detector are turned to face each other to measure the source luminance (radiance).
BRDF Measurements
Specifications on how to make BRDF measurements are not included in the IDMS; more research was needed at the time of its compilation. The apparatus employed here had a medium resolution of 0.6° and a specular configuration angle of 3°. It used a converging source (white LED) and a quasi-photopic detector.6 The source iris was approximately 1 mm in diameter and was focused onto the detector iris of 6.3 mm in diameter. (The source had a beam diameter of 48 mm and a 300-mm focal-length lens resulting in a focal ratio of f/6.3.) We only provided the horizontal in-plane BRDF from 3° away from the display normal out to 70°. The source was fixed at –3° relative to the display normal and the detector was rotated about the display center. The source incident flux was determined by placing a calibrated RG-1000 black glass near the screen and repositioning the detector for the displacement from the display surface at the ±3° configuration. The resulting BRDF profiles illustrate the matrix scatter, especially in the Samsung OLED (see Fig. 9).
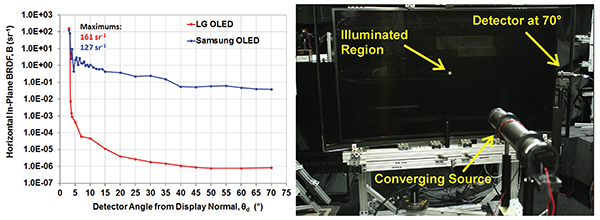
Fig. 9: BRDF results for both OLED displays appear in the graph at left. The photograph on the right shows the BRDF measurement configuration with the detector at 70° from the display normal. The 48-mm-diameter converging source is 3° to the left of normal and illuminates a small region on the display. The detector measures the specular component when at 3° to the right of the display normal and the scattered light at angles greater than 3° away from the normal.
A Need for New Measurements
Because we observed a darkening of the OLEDs in the Samsung display when it was subjected to very large illuminances, the assumption of linearity and superposition in reflection measurements may not always be true. Linear superposition was a fundamental assumption in the reflection chapter of the IDMS (see 11.1.1). This singular darkening result will require a complete reconsideration of the material in Chapter 11 on reflection in the IDMS document and especially the section on ambient contrast, § 11.9. We did not imagine this would ever happen! Understand that this darkening observation is not a criticism of the Samsung display; it just means that we have encountered a new property in the measurement of reflections that was not previously anticipated. We will have to document a procedure to detect such a property and invent a procedure to make such reflection measurements on non-black patterns in the future.
We have seen how the LG OLED display has benefitted from an anti-reflection front surface and good control of matrix scatter. These results also show that the Samsung OLED has a very wide matrix scatter distribution without any anti-reflection treatment; the matrix scatter especially interferes with good control of reflection properties, particularly for isolated sources in the surround that can create a band of reflected light in the display.
While these are beautiful displays that have zero-luminance blacks, reflections from the room reduce the available contrast because of adding light to those wonderful blacks. Thus, in one simulation we found contrasts from 400 in the Samsung OLED to 1058 in the LG OLED coming from illumination conditions for a living room. However, these contrasts are better than displays that exhibit a non-zero black where the resulting contrasts would be even less for the same living-room environment: For example, a display with a white luminance LW = 400 cd/m2 and a black luminance LK = 1 cd/m2 (giving a darkroom contrast of 400:1) having the same reflection properties as the LG OLED would exhibit a contrast of CA′ = 290 for those same living-room conditions where the LG OLED exhibited a contrast of CA′ = 1058. These OLED displays may well present a better overall image appearance than others under moderate- to-high ambient conditions because of their absolute blacks, but for best viewing experience we will enjoy them most in a very dark theater environment.
References
1E. F. Kelley, “Considering Color Performance in Curved OLED TVs,” Information Display 29, No. 6, 13 (2013).
2This work was partially funded by LG Display Co., Ltd. Their contribution to our effort is again gratefully acknowledged.
3M. E. Becker, “Display reflectance: Basics, measurement, and rating,” J. Soc. Info. Display 14, 1003–1017 (doi: 10.1889/1.2393025) (2006).
4As in the previous article, we make repeated reference to the Information Display Measurements Standard (IDMS), prepared by the International Display
Metrology Committee of the Society of Information display. The PDF version is available without charge; see http://icdm-sid.org/.
5Disclaimer: The apparatus pictured or described herein are identified only for the purpose of complete technical description: The signals are provided from a computer using an NVIDIA GeForce GTX 570 board with an HDMI (high-definition multimedia interface) output. The signal output quality is checked using computer monitors to assure that what is delivered to the TVs is correct without artifacts. The spectroradiometric measurements are made with a Photo Research PR-730 spectroradiometer. All measurements are made in a quality darkroom.
6See, ASTM Standards on Color and Appearance Measurement, 6th ed., E 167-96, “Standard Practice for Goniophotometry of Objects and Materials,” pp. 264–267 (2000) (withdrawn 2005). Also, see E 2387 – 05, “Standard Practice for Goniometric Optical Scatter Measurements,” in the 8th ed. •
Ed Kelley is a consulting physicist with Keltek LLC in Longmont, Colorado. He can be reached at ed@keltekresearch.com.