Rendering of Detail in Televisions
Rendering of Detail in Televisions
One of the creators of the IDMS (Information Display Measurements Standard) takes the measure of the very latest curved OLED TVs compared to LCD TVs. In this third article in a series, he looks at how well these displays render detail and at their resolution performance.
by Edward F. Kelley
IN the previous two articles in this series, we limited ourselves to comparing the two curved AMOLED televisions that had recently been released: the LG 55EA9800 and the Samsung KN55S9CAFXZA. For the sake of comparison, we are now adding two LCD TVs with LED backlighting to the mix: the Samsung UN55F7100AF and the LG 55LB7200. All are 55-in displays.
As in the previous articles, only one display from each manufacturer was measured, so a statistical sampling is not provided.1,2 Please keep in mind that manufacturing details can change as newer displays are released with different properties. Additionally, using the Internet to download new operating software may also produce different characteristics depending upon the software modifications. Last, just as in the previous articles, we continue to make parenthetical references to the IDMS document where more information can be found.3
To test how these displays render detail (in 2-D only for this article), we needed certain setup conditions. Figure 1 shows a test pattern that we employed for some of our testing. (This may eventually be available on the www.icdm-sid.org site.) This pattern provides red-green-blue-white (RGBW) detail at a small average pixel level (APL) centered on a 3 × 3 matrix with complementary colors included. The tested displays needed to be set up with a 1:1 correspondence between the input-signal pixels and the displayed pixels, otherwise the rendering of this type of pattern would be compromised. Additionally, any sensitivity to ambient lighting had to be turned off, and any dimming features were likewise turned off as much as possible. Other than these special settings, our measurements used factory default settings for each display except where noted for the control of sharpening. We considered the standard modes and the theater modes (Movie and THX Cinema) for these displays.
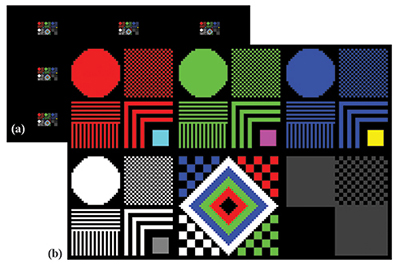
Fig. 1: Shown is a test pattern with nine elements. The RGB pattern element (b) is 160 px × 106 px to keep the APL low. The disks and 1 × 1 checkerboards are 24 px wide or high. The large white diamond is 49 px high and provides single-stairstep pixel regions; its RGBW bands are 5 px wide and high, and it is surrounded by a 4 × 4 pixel checkerboard. The bottom right cluster has a dark-gray background (63/255) for observing sharpening artifacts on a 2 × 2 checkerboard.
Photography of Displayed Detail
The photography of display screens can be tricky. Even when close to the TV or computer monitor, the eye will generally not resolve the subpixel detail but will see the pixels as complete patches of light, and it was necessary to capture such a rendering in our photography as much as possible. The separation between the pixels will be visible because usually there is not a 100% fill factor for the pixels (see IDMS §7.4). To avoid moiré and render the photograph approximately as the eye sees it usually requires a large f-number (small effective aperture) and a reasonable distance from the screen.4
One of the problems with the photography of film-patterned-retarder (FPR) displays such as the LG displays used here is that some cameras will render a slightly larger gap between every two rows of pixels because of their sensitivity to circularly polarized light, presumably because of the autofocusing mechanism. Should this happen it can be eliminated using a linear polarizer in front of the camera lens with its polarization axis oriented at 45° with respect to the horizontal or vertical in order to minimize any color shift (see Fig. 2). The eye sees evenly spaced rows on the display as in Fig. 2(b). We used a Canon EOS T3i to photograph the screen images.5
(a)
(b)
Fig. 2: These pixel-resolved photographs show the LG OLED FPR display using a linear polarizer to eliminate the camera-induced uneven row spacing. The left photograph (a) shows a larger row spacing between every two rows, which is not observed by the eye. The right photograph (b) is taken with a linear polarizer with its axis at 45° (relative to the vertical or horizontal) in front of the camera lens; this eliminates the camera’s sensitivity to circularly polarized light so that the pixel rows appear evenly spaced as the eye sees them (55 mm lens, f/22, at 1.8 screen heights).
If we want to visually compare a displayed image with the input image, it can be difficult using our eyes: We see a pixelated image on the display with less
than a 100% fill factor, but the input image is often rendered without a fill factor, such as when just one element is examined on a computer monitor, as in Fig. 1. To make the input image appear more like the displayed image, we can magnify it and introduce a grid between the pixels (see Fig. 3). The original image is sent to the display unmodified as in Fig. 1, but to visually compare the displayed image with the photograph of the displayed image we needed an added grid simulating less than a 100% pixel fill factor. If we do not do this, we can be sometimes be fooled into thinking that the display is not doing its job correctly.
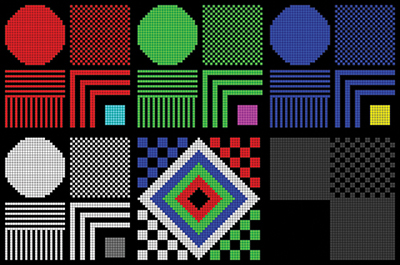
Fig. 3: An artificial addition of a pixel grid is used for visual comparisons with photographs of the displayed pattern. The unmodified image is sent to the display. The addition of the grid is only for visual comparisons. The pattern element is expanded 30×. Grid lines are added of a specified width (in this case 5 px); then the result is reduced to a final magnification of 5× its original size using a bilinear interpolation in MATLAB.
To attempt to understand reasons for any irregularities in the observed pattern, we needed to examine the details of the pixels and their subpixel contributions. We captured subpixel detail using a set of three extension tubes with a 55-mm lens set at f/8 placed approximately 4.5 cm from the display surface (see Fig. 4).
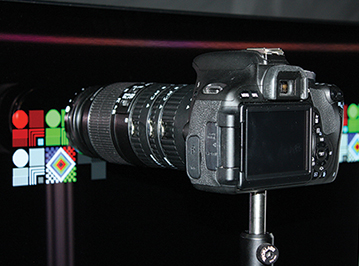
Fig. 4: The camera was set up to capture subpixel detail.
Detail Rendering
We show photographs of our pattern element for the OLED displays in Figs. 5–7. Figure 5 shows the Samsung OLED with its default sharpening of 50. Figure 6 shows the same Samsung OLED with its sharpening turned to zero. Figure 7 shows the LG OLED with its default sharpening of 20. In all the figures, we show the subpixel structure in the inset photographs at the top for the solid green color and the 1 × 1 green checkerboard. In all these three figures note that the white 1 × 1 vertical and horizontal grilles (bottom left corner) are clearly resolved, indicating full resolution of the displays, as expected. Also note in these three figures that the vertical grilles for the red and blue are smeared. This is presumably a result of the chroma sub-sampling encountered in the
processing in TVs. Because of the usually large viewing distances for TVs, the eye will not resolve the red and especially the blue for the luminances involved, so there is no reason to spend the money to perfectly recreate all the colors at full resolution. Computer monitors should render such red and blue grilles perfectly, but that will not be the case for most TVs. Thus, many TVs for entertainment will not serve perfectly for a close-up computer monitor, but they will render video imagery and casual computer images adequately for the pleasure of the observer. In all three figures, the blue pixels are overexposed and appear to be exhibiting color fade, but such is not the case.
In Fig. 5 with the Samsung OLED sharpness setting of 50, the green 1 × 1 checkerboard appears more faded, pale, and less saturated than the red compared to their solid colors. Also note the large increase in the luminance of the 2 × 2 dark-gray checkerboard compared to the solid color. Definitely the sharpening interferes with the accurate rendering of fine detail, as expected. However, even with the sharpening turned off (to zero), Fig. 6 of the Samsung OLED continues to show a color fading, especially in the green 1 × 1 checkerboard, whereas the 2 × 2 gray checkerboard shows no sharpening artifacts. The inset photographs show the contribution of red and blue subpixels to what would be expected to be only green subpixels. The red and blue subpixels are less intense in the inset photograph of the 1 × 1 green checkerboard in Fig. 6 with the sharpening turned off, but that may be difficult to see in the photographs.
Figure 7 shows the LG OLED with no discernable sharpening artifact in the 2 × 2 gray checkerboard, despite a sharpening setting of 20. However, there is a small color fade in the green that cannot be seen in the photograph of the pattern element and cannot be discerned by the eye, but is quite visible in the inset photographs comparing the subpixel illumination in the solid green to the 1 × 1 green checkerboard. We would assume that such activation of unrelated subpixels is a manifestation of the processing algorithms used by these displays. Note some of the spillover of the white and green into the black regions of the 4 × 4 checkerboards at the bottom center of Fig. 7, which does not occur for the red or blue – presumably these are also artifacts of processing.
Upon careful examination of the magenta square in these three figures, note that there is a red and blue edge on the magenta square. In the Samsung OLED, these edges appear on the top and bottom because of the vertically aligned subpixels. In the LG OLED, the edges appear on the left and right because of the horizontally aligned subpixels.
How the sharpening artifacts can manifest themselves is illustrated in Fig. 8 for the Samsung OLED display. The large photograph is what appears on the screen with sharpening turned off. The two inset pictures compare the default sharpening of 50 (left inset) with the sharpening of zero (right inset). When there is such fine detail, the sharpening will cause color fade. However, as we have seen, color fade can occur when there are no sharpening artifacts.
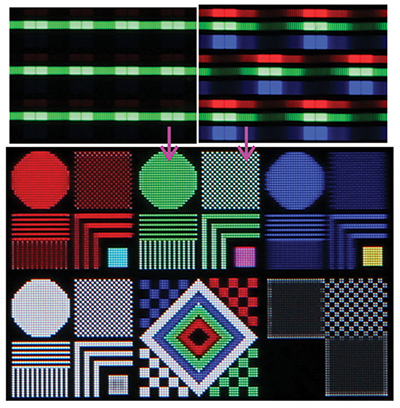
Fig. 5: This pattern element photograph shows a Samsung OLED in standard mode with its default sharpening of 50. The inset photographs above the photograph of the pattern element show the subpixel structure of the solid green and the 1 × 1 green checkerboard.
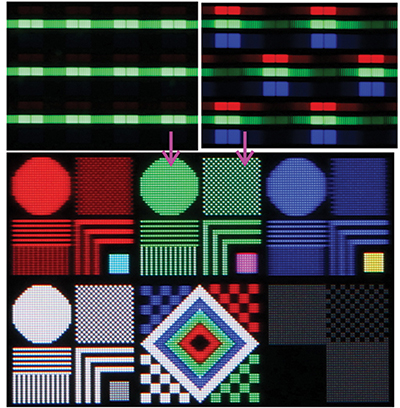
Fig. 6: This pattern element photograph of a Samsung OLED in standard mode shows the sharpening changed to zero. The inset photographs above the photograph of the pattern element show the subpixel structure of the solid green and the 1 × 1 green checkerboard. Even with sharpening turned off, there remains some visible color fade.
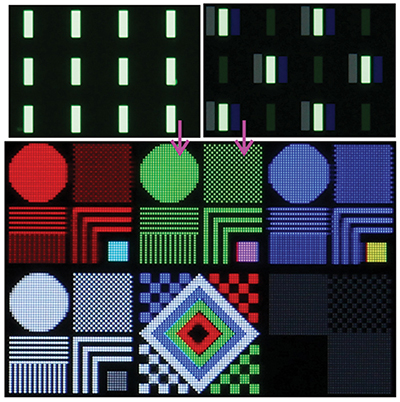
Fig. 7: This pattern element photograph shows an LG OLED in standard mode with its default sharpening of 20. The inset photographs above the photograph of the pattern element show the subpixel structure of the solid green and the 1 × 1 green checkerboard.
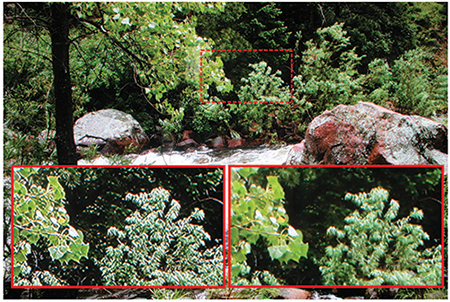
Fig. 8: Color fade can be a result of sharpening. The left inset magnified cut is the Samsung OLED with its default sharpening setting of 50. The right inset is the same display with the sharpening reduced to zero.
Measurement Results
Although measurements of our pattern element are possible, it would require measuring the screen at different but nearby points, and there is always a concern in comparing different measurement points on the screen. To measure the various components found in the pattern element, we measured each component of the pattern as a centered 10% area of the screen (see Fig. 9). The spectroradiometer is placed at approximately 1.8 screen height and employs a 2° aperture (approximate measurement-field angle). It is focused on the center of the rectangle, and then the patterns to be measured are cycled through the collection. Careful attention is paid to any changes in the black level because of a pattern change. If any changes are observed, then the black background is replaced with a 1% starfield background.
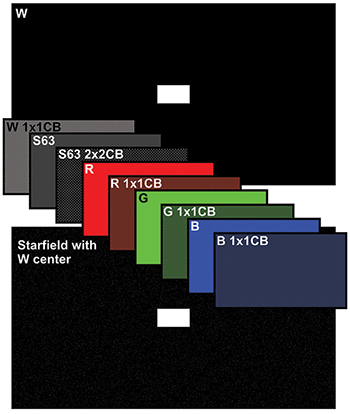
Fig. 9: Rectangles of colors and pixel checkerboards (CB) at 10% of the screen size are placed at the center of the screen to obtain the sharpening metric and color-fade metrics. If darkening of the screen cannot be adequately controlled through the display settings, then a 1% starfield pattern is used instead of a black background and the 10% rectangles are placed at the center of that starfield.
We want a metric that will call attention to the effects of sharpening, which is often seen by brightening the edges of lines and a desaturation of colors in detail. Even when sharpening is not present, we also want a metric to detect any color changes in detail because of whatever processing is being done on the image that results in a change in color of detail. We can define two metrics to characterize the sharpening and the color fades (see text boxes). The sharpening is based upon how much of the white is added to the 2 × 2 gray (shade 63/255) checkerboard compared to the white level and is expressed in the percent of white. The color-fade metric is based on comparing the chromaticity coordinates of the solid color to that of the 1 × 1 checkerboard of that same color.
Sharpening Metric (Proposed)
Let LW be the luminance of the white box, LK be the luminance of black, LS63 be the luminance of the gray box (“S63” is for shade 63/255), and LS63 2x2 be the luminance of the gray 2 × 2 checkerboard. We propose a sharpening metric S based upon the percentage of white added to the 2 × 2 gray checkerboard:
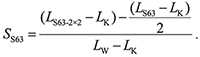
Here, we subtract off half the net luminance of the solid gray luminance from the net luminance of the gray checkerboard and divide it by the net white luminance. |
Color-Fade Metric (Proposed)
For any color Q = R, G, or B, let (u'Q, v'Q) be the chromaticity coordinates of the solid color, and let (u'Q1×1, v'Q1×1) be the chromaticity coordinates of the 1 × 1 checkerboard of the same color. We propose that the Euclidian separation of the two colors characterize the color fade:

Here, we are using the 1976 CIE (Com-mission Internationale de l’Eclairage [International Commission on Illumination]) chromaticity coordinates. |
The results appear in Table 1. It can be hard to see a few percent in the SS63 sharpening metric using our test pattern. However, sharpening artifacts of 10% and larger are quite visible. We highlight color fades greater than 0.04 that might be distinguishable on different parts of the display surface (see Appendix B1.2 Colorimetry in IDMS, where it discusses that a Δu'v' of 0.04 can serve as the limit for separated colors, whereas 0.004 is the limit for adjacent colors). The table includes both the standard and theater mode results. In Fig. 10, we show the chromaticity coordinates of the data represented in Table 1 for the standard modes on all displays. The data points that are farthest from the white point are the solid colors, the data that extend farthest toward the white-point area are for the default sharpening setting, and the data points between them are where sharpening has been set to zero. The distance between the outer points and the more central points indicates the color fade. For all these displays there is not much color fade in the blue. Because the color fade for all these displays in the theater modes is small, we do not show a similar plot for the theater modes.
Table 1: Sharpening and color-fade measurement results.
\
Display |
Mode |
Sharpening Setting |
Sharpening Metric, SS63 |
Red Color Fade Δu'v'Rfade |
Green Color Fade Δu'v'Gfade |
Blue Color Fade Δu'v'Bfade |
|
Standard |
50 (default) |
16.9% |
0.101 |
0.136 |
0.020 |
Samsung OLED |
Standard |
0 |
-0.3% |
0.034 |
0.091 |
0.009 |
|
Movie |
0 |
-0.2% |
0.026 |
0.059 |
0.003 |
|
Standard |
20 (default) |
0.1% |
0.017 |
0.017 |
0.002 |
LG OLED |
Standard |
0 |
-0.1% |
0.016 |
0.017 |
0.002 |
|
THX Cinema |
0 (default) |
0.0% |
0.024 |
0.029 |
0.007 |
|
Standard |
50 (default) |
10.94% |
0.094 |
0.055 |
0.008 |
Samsung LCD |
Standard |
0 |
-0.27% |
0.024 |
0.030 |
0.012 |
|
Movie |
20 (default) |
1.10% |
0.019 |
0.030 |
0.006 |
|
Standard |
20 (default) |
1.01% |
0.042 |
0.079 |
0.003 |
LG LCD |
Standard |
0 |
-0.26% |
0.031 |
0.063 |
0.002 |
|
Cinema |
10 (default) |
-0.37% |
0.039 |
0.049 |
0.007 |
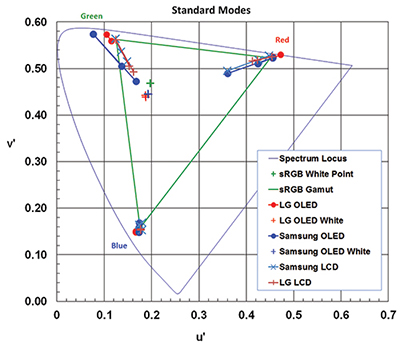
Fig. 10: Color-fade characteristics of all displays in standard mode are shown on the color diagram. The coordinates of the solid colors define the gamut and the 1 × 1 checkerboard coordinates are shown with their default settings and with sharpening turned off.
We have tried to present two new metrics that will help characterize displays: a sharpening metric and a color-fade metric. We have seen that sharpening can produce a color fading in detailed imagery, but we have also seen that color fade can occur when there are no sharpening artifacts present. It is important to keep in mind that these are all beautiful displays – especially the OLED displays. We develop metrics to characterize displays. Such metrics not only help users evaluate displays, but also serve to help manufacturers evaluate their own products. How these two metrics appear in the upcoming version 2 of the IDMS may depend upon further discussions and further research, but they will be submitted for consideration.
As a final aside: While measuring these displays with the LCDs next to the OLED TVs, the beautiful deep blacks of the OLED displays were clearly evident; also evident is how the OLED displays show significantly fewer viewing-angle changes. The author would like to congratulate the companies that developed the OLED displays; they are simply beautiful!
References
1E. F. Kelley, “Considering Color Performance in Curved OLED TVs,” Information Display 29, 6 (2013); and “Considering Reflection Performance in Curved OLED TVs,” Information Display 30, 3 (2014).
2This work was partially funded by LG Display Co., Ltd. Their contribution to our effort is again gratefully acknowledged.
3Information Display Measurements Standard (IDMS), prepared by the International Display Metrology Committee of the Society of Information display. The PDF version is available without charge; see, http://icdm-sid.org/.
4E. F. Kelley, “Photography of Display Surfaces Using Consumer Cameras – Three Regimes and Tristimulus Imagery,” 2013 SID International Symposium Digest of Technical Papers, Society for Information Display, Invited paper 24.1, pp. 283–286, Vancouver, Canada (May 2013).
5Disclaimer: The apparatus described herein are identified only for the purpose of complete technical description: The signals are provided from a computer using an NVIDIA GeForce GTX 760 board with an HDMI (high-definition multimedia interface) output. Signal integrity was tested using a computer monitor at the end of the HDMI cable inserted into the TV. The camera used for the detailed photography was a Canon T3i. Spectroradiometric measurements were made by a Photo Research PR-730. All photographs and measurements were made in a quality darkroom. •
Ed Kelley is a consulting physicist with Keltek LLC in Longmont, Colorado. He can be reached at ed@keltekresearch.com.