OLEDs with Candle-Like Emission
OLEDs with Candle-Like Emission
Candle-like organic light-emitting diodes (OLEDs) emit much less blue light than other non-incandescent light sources. This type of OLED is characterized by low correlated color temperature (CCT), chromaticity tunability, and low melatonin (MLT) suppression. The light evokes a sense of warmth and creates a pleasant and calming ambience with its high-quality diffuse orange-red emission.
by Jwo-Huei Jou, Meenu Singh, Yi-Fang Tsai, Hui-Huan Yu, Szu-Hao Chen, Sheng-Hsu Shih, and Shang-Chih Lin
THE medical community has been sounding alarms with increasing frequency about the health hazards of blue light. An International Energy Agency report from 2014 stated that blue and cool-white light-emitting diodes (LEDs) could damage light-sensitive tissues in eyes and even lead to blindness.1,2 Numerous medical studies have also reported that intense blue or white light may cause circadian disruptions3-5 and sleep disorders.6 Notably, Stevens et al. discovered that electric light at night was at least in part a factor in causing breast cancer through the mechanism of suppressing the oncostatic hormone melatonin.7,8 Specifically, the rate of breast-cancer occurrence was 73% higher in females in communities with the brightest light at night than those in the darkest comparative environments.9 Outside the human sphere, the International Dark-Sky Association reports that light pollution at night disrupts the life-sustaining behavior of many nocturnal animals such as birds, amphibians, and insects, causing damage to the ecosystem.10 Other potentially deleterious effects from short-wavelength light, especially that from LEDs, include the discoloration of oil paintings in museums, including works by Van Gogh and Cézanne, as reported in 2013.11,12
A New Light-Quality Metric
It should now be clear that we need a light source that is reliably benign in terms of the health of both people and the environment while providing quality illumination. Even the U.S. Department of Energy, unwavering in its focus on energy-consumption reduction, has acknowledged the health effects of light and the need for new metrics that describe improvements in “health and productivity” in its 2013 Multi-Year Program Plan.13 That said, no currently available lighting-quality metric measures the impact of lighting on human health; instead, they focus on the color point and color rendering of light sources (see the article, “Light for Life: Emerging Opportunities and Challenges for Using Light to
Influence Well Being” in this issue).
One such well-known metric is the color-rendering index (CRI), which describes the color fidelity of various samples illuminated by said light source vs. a black-body radiator of the same correlated color temperature (CCT). It is worth noting that a black-body radiator will by definition have a CRI of 100, the maximum value. At CCTs of 2000K or lower, black-body radiation has very little spectral content in the blue region [e.g., Fig. 3(a)]. Thus, a light source with a spectral power distribution (SPD) similar to that of a black-body radiator at low CCTs will simultaneously have high color rendering and very low emission of hazardous blue light.
The authors’ group at National Tsing-Hua University in Taiwan developed the spectrum resemblance index (SRI) as a metric for the percentage similarity between a
given light source and its corresponding black-body radiator at the same CCT14:
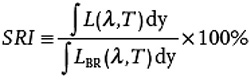
where LBR(λ, T) is the luminance spectrum of the black-body radiator at the specific CCT and L(λ,T) is the overlapping area between the luminance spectra of the light source under investigation and its corresponding black-body radiator.14 Luminance instead of power spectra are used since they are more relevant to gauging human visual responses. SRI can be calculated for any light source and will range between 0 and 100 for total non-overlap or identity with the black-body radiator.
Figure 1 shows the power and luminance spectra for various light sources as well as the
calculated SRI. For high-intensity-discharge (HID) lamps with “spikey” spectra, the SRIs are in the 60s or even as low as the 30s. The cool fluorescent lamp is only slightly better with an SRI of 78. Thus far, SRI has done a good job summarizing the resemblance to black-body radiation in a single metric. In the modern white LEDs and OLEDs studied, the spectra are substantially more continuous. They arise in the LEDs due to the broad phosphor peak(s) and in OLEDs due to the intrinsically broad emission of the organic chromophores. The calculated SRIs are all in the 90s and thus enable much discrimination between the sources. There remains a need to develop a light quality more sensitive to the blue part of the spectrum. In particular, any such metric should also recognize the need for circadian stimulation (or the lack thereof) during different times of the day, which remains an active area of research.15
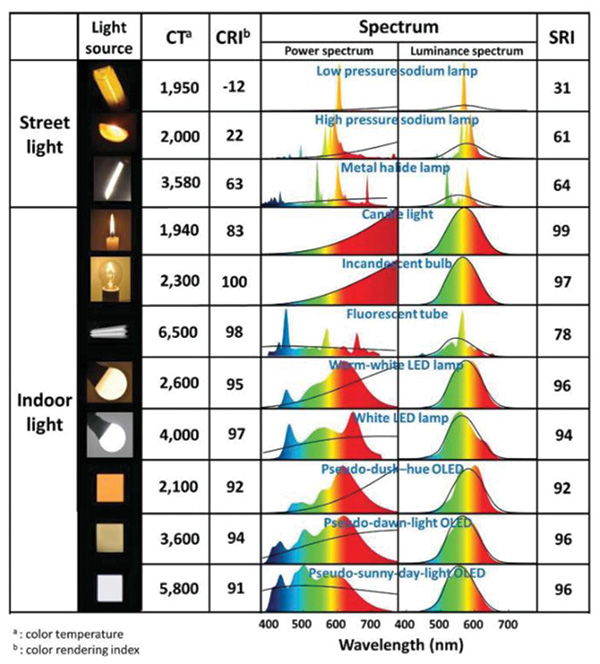
Fig. 1: Various light sources are shown along with their power and luminance spectra, SRI, CRI, and CCT.16 The CRI reported here is compared with a 2860K reference rather than a
black-body radiator of the same CCT.
OLEDs with Candle-Like Emission
Candles were the main source of artificial illumination for mankind for thousands of years. Even today, candlelight evokes a sense of warmth and creates a pleasant and calming ambience. The flame itself is a complex source composed of regions of different color temperatures where we use the brightest spot at 1914K to represent the whole (Fig. 2).17 The candle spectrum has inherent low bluespectral content that minimizes MLT suppression in the evening. However, the natural candle is an extremely inefficient light source, with a total efficacy of 0.1–0.3 lm/W, not to mention the other shortcomings of a combustible source. On the other hand, a solid-state light source that matches the visible spectrum of a candle holds great promise.
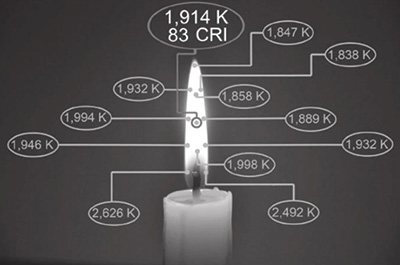
Fig. 2: Different color temperatures are shown at different flame positions. The color temperatures vary from 1847 to 2626K, with the brightest spot at 1914K.17 The CRI reported here is compared with a 2860K reference rather than a black-body radiator of the same CCT. Candlelight should have a CRI of 100 since it is a black-body radiator.
Most white OLEDs for lighting contain three emitters: red, green, and blue (RGB), in either two-stack (RG,B) or three-stack (R,G,B) architectures. Over the years, our group fabricated a number of warm white OLEDs with two- or three-emitter systems.18-22 However, even with three broad emitters, there can still be a sizable gap in the resulting spectrum. In 2012, our group was the first to develop a four-emitter OLED containing red, yellow, green, and sky-blue phosphorescent emitters (Fig. 3).17 Adjusting device parameters allowed a CCT range of 1918–3000K to be accessed, at 1000 cd/m2. An optimized device had a broad spectrum resembling that of a black-body emitter and achieved 19 lm/W, a higher CRI of 93 at a CCT of approximately 2000K (Fig. 4).17 It can be seen from Fig. 4(b) that the device spectrum still deviated appreciably from that of a black-body radiator, which resulted in an SRI of 80.
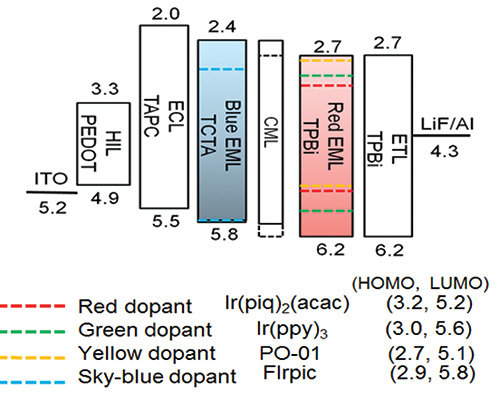
Fig. 3: This schematic illustration of the candlelight OLED composes four black-body-radiation complementary dyes; namely, red, yellow, green, and sky-blue, dispersed in two emissive layers separated by a nano-interlayer to harvest the ultimate color-rendering index and device efficacy.17
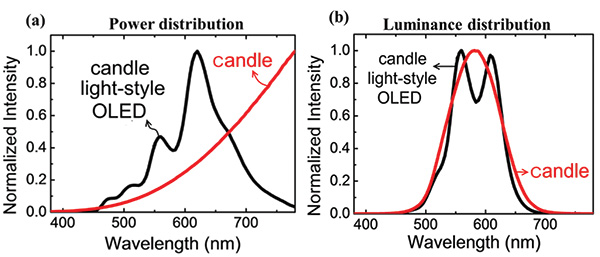
Fig. 4: These two charts compare the (a) spectral power and (b) luminosity distributions of a candlelight OLED with a CCT of 2000K with a candle.17
Pseudo-Natural Light
We continue to develop OLEDs with many (>3) emitters with ever-increasing spectrum tenability and CCT range, culminating in devices with as many as six emitters in three different emitter layers (EMLs) [Fig. 5(a)].16,23,24 With so many emitters, the spectra are largely continuous such that we coined the term “pseudo-natural light” for these OLEDs (see bottom of Fig. 1). Figure 5(b) depicts the simulated and actual luminance spectra of devices at 2100, 3600, and 5800K. The SRI for these devices is well into the 90s, indicating a high resemblance to black-body radiation.
With six emitters – deep-red, orange-red, yellow, green, sky-blue, and deep-blue – it is possible to produce white light and achieve good SRI along the Planckian locus from 1100K and higher. For a more-detailed discussion on color mixing, see “Pseudo-Natural Light for Displays and Lighting” in the journal of Advanced Optical Materials.16
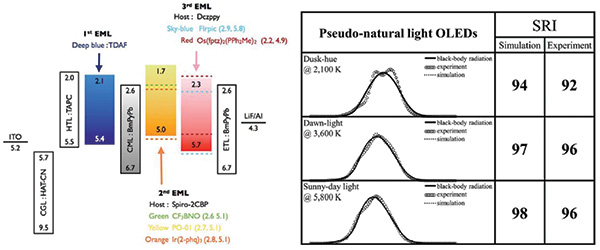
Fig. 5: (a) At left are the energy-level diagram and layer structure of the six-emitter pseudo-natural-light OLED. (b) At right are the simulated and actual luminance spectra and SRI for the three different CCTs: dusk hue, 2100K; dawn light, 3600K; and sunny day light, 5800K.
Low MLT Suppression
Kozaki et al. reported that exposure to 200-lx illuminance from a 5000K fluorescent lamp for 90 minutes between 1:00 to 2:30 am suppressed MLT secretion by 78%. The suppression was 50% for 3000K and 17% for 2300K lamps.25 According to this study, we expected exposure to the candlelight OLED of 1920K to result in a MLT suppression of less than 10% under similar conditions. However, in a recent study conducted by our own group, exposure to a candlelight OLED at 100 lx for 90 minutes from 2:00 to 3:30 am resulted in MLT suppression of 33% vs. 64% for a 5710K fluorescent lamp.26 While the candlelight OLED led to significantly less-measurable MLT suppression, it is clear there is much work to be done to establish a qualitative understanding of the relationship and all the variables involved.
Commercialization
Commercialization of the candlelight OLED started at the end of 2014 by Wisechip Semiconductor of Taiwan.27 The company is currently producing panels of 10 × 10 cm2 in size. The first installation was in street lights for an aboriginal group – the Atayal Tribe in Smangus, Taiwan. The panels were deployed in lamp shades made of old wood (Fig. 6). The tribe required that the light sources be both human- and eco-friendly.
Our research team hopes that these multi-emitter candlelight OLEDs will eventually be used in a range of indoor and outdoor luminaires. These OLEDs have spectra that resemble low CCT black-body radiators characterized by good color rendering and much reduced blue spectral content, which in turn leads to reduced MLT suppression. It is reasonable to hope that such lighting will be conducive to human and ecological health.
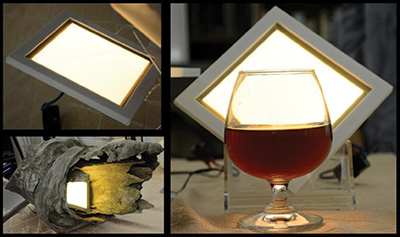
Fig. 6: These candlelight OLED panels were first produced for the Atayal Indigenous Taiwanese people who were without electricity until 1979. They had been using CFL-based street lights but rejected a suggestion by the government to install LED lights.
Acknowledgment
This work was financially supported by the Ministry of Science and Technology through grants 104-2119-M-007-012 and 103-2923-E-007-003-MY3 and by the Ministry of Economic Affairs through grant 102-EC-17-A-07-S1-181.
References
1International Energy Agency final report on potential health issues on SSL (September 2014).
2R. Reilly; http://www.dailymail.co.uk/health/article-2324325/Do-environmentally-friendly-LED-lights-cause-BLINDNESS.html
3S. M. Pauley, “Lighting for the human circadian clock: Recent research indicates that lighting has become a public health issue,” Med. Hypotheses 63, 588–596 (2004).
4P. R. Mills, S. C. Tomkins, and L. J. M. Schlangen, “The effect of high correlated colour temperature office lighting on employee
wellbeing and work performance,” J. Circadian Rhythm 5, 1–9 (2007).
5M. Sato, T. Sakaguchi, and T. Morita, “The effects of exposure in the morning to light of different color temperatures
on the behavior of core temperature and melatonin secretion in humans,” Biol. Rhythm. Res. 36, 287–292 (2005).
6J. Arendt, “Melatonin, circadian rhythms, and sleep,” New Engl. J. Med. 343, No. 15, 1114–1116 (2000).
7R. G. Stevens, G. C. Brainard, D. E. Blask, S. W. Lockley, and M. E. Motta, “Breast cancer and circadian disruption from electric lighting in the modern world,” CA Cancer J. Clin. 64, No. 3, 207–218 (2014).
8S. Davis, D. K. Mirick, and R. G. Stevens, “Night-shift work, light at night, and risk of breast cancer,” J. Natl. Cancer Inst. 93, 1557–1562 (2001).
9I. Kloog, A. Haim, R. G. Stevens, M. Barchanade, and B. A. Portnov, “Light at Night Co-Distributes with Incident Breast but Not Lung Cancer in the Female Population of Israel,” Chronobiology Intl. 25, 65–81 (2008).
10http://www.darksky.org/
11http://www.vangogh.ua.ac.be/
12L. Monico, K. Janssens, C. M iliani, B. G. Brunetti, M. Vagnini, F. Vanmeert, G. Falkenberg, A. Abakumov, Y. Lu, H. Tian, J. Verbeeck, M. Radepont, M. Cotte, E. Hendriks, M. Geldof, L. van der Loeff, J. Salvant, and M. Menu, “Degradation Process of Lead Chromate in Paintings by Vincent van Gogh Studied by Means of Spectromicroscopic Methods. 3. Synthesis, Characterization, and Detection of Different Crystal Forms of the Chrome Yellow Pigment, S. Anal. Chem. 85(2), 851–859 (2013).
13Solid-State Lighting Research and Development: Multi-Year Program Plan, Office of Energy Efficiency and Renewable Energy, U.S. Department of Energy, Washington, DC (April 2013), p. 52.
14J. H. Jou, K. Y. Chou, F. C. Yang, A. Agrawal, S. Z. Chen, J. R. Tseng, C. C. Lin, P. W. Chen, K. T. Wong, and Y. Chi, “A universal, easy-to-apply light-quality index based on natural light spectrum resemblance,” Appl. Phys. Lett. 104, 203304 (2014).
15M. E. J. Bouwmans, E. H. Bos, S. H. Booij, M. van Faassen, A. J. Oldehinkel, P. de Jonge, “Intra- and inter-individual variability of longitudinal daytime melatonin secretion patterns in depressed and non-depressed individuals,” Chronobiology International 32(3), 441–446 (2015).
16J. H. Jou, K.Y. Chou, F. C. Yang, C. H. Hsieh, S. Kumar, A. Agrawal, S. Z. Chen, T. H. Li , and H. H. Yu, “Pseudo-Natural Light for Displays and Lighting,” Adv. Optical Mater. 3, 95–102 (2015).
17J. H. Jou, C.Y. Hsieh, J. R. Tseng, S. H. Peng, Y. C. Jou, J. H. Hong, S. M. Shen, M. C. Tang, P. C. Chen, and C. H. Lin, “Candle-Light-Style Organic Light-Emitting Diodes,” Advanced Functional Materials 23(21), 2750–2755 (2013).
18J. H. Jou, S. H. Chen, S. M. Shen, Y. C. Jou, C. H. Lin, S. H. Peng, S. P Hsia, C. W. Wang, C. C. Chen, and C. C. Wang, “High efficiency low-color-temperature organic light-emitting diodes with a blend interlayer,” J. Mater. Chem 21, 17850 (2011).
19J. H. Jou, P. Y. Hwang, W. B. Wang, C. W. Lin, Y. C. Jou, Y. L. Chen, J. J. Shyue, S. M. Shen, and S. Z. Chen, “High-efficiency low color temperature organic light emitting diodes with solution-processed emissive layer,” Org. Electron. 13, 899–904 (2012).
20J. H. Jou, M. C. Tang, P. C. Chen, Y. S. Wang, S. M. Shen, B.R. Chen, C. H. Lin, W. B. Wang, S. H. Chen, C.T. Chen, F.Y. Tsai, C. W. Wang, C. C. Chen, and C. C. Wang, “Organic light-emitting diode-based plausibly physiologically-friendly low color-temperature night light,” Org. Electron. 13, No. 8, 1349–1355 (2012).
21J. H. Jou, “A festive switch: OLEDs instead of candles?”; http://www.materialsviews.com/a-festive-switch-oleds-instead-of-candles
22J. H. Jou, S. Kumar, C. C. An, M. Singh, H. H. Yu, C.Y. Hsieh, Y. X. Lin, C. F. Sung, and C.W.Wang, “Enabling a blue-hazard free general lighting based on candle light-style OLED,” Optics Express A577, 23, 11; DOI:10.1364/OE.23.00A576.
23J. H. Jou, P. W. Chen, Y. L. Chen, Y. C. Jou, J. R. Tseng, R. Z. Wu, C. Y. Hsieh, Y. C. Hsieh, P. Joers, S. H. Chen, Y. S. Wang, F. C. Tung, C. C. Chen, and C. C. Wang, “OLEDs with chromaticity tunable between duskhue and candle-light,” Org. Electron. 14, No. 1, 47–54 (2013).
24J. H. Jou, R. Z. Wu, H. H. Yu, C. J. Li, Y. C. Jou, S. H. Peng, Y. L. Chen, C. T. Chen, S. M. Shen, P. Joers, and C. Y. Hsieh, “Artificial dusk-light based on organic light emitting diodes,” ACS Photonics 1, No. 1, 27–31 (2014).
25T. Kozaki, S. Koga, N. Toda, H. Noguchi, and A. Yasukouchi, “Effects of short wavelength control in polychromatic light sources on nocturnal melatonin secretion,” Neuroscience Letters 439, 256–259 (2008).
26Y. C. Jou et al., “Ambient light effect on melatonin secretion,” (in preparation).
27(a) http://www.oled-info.com/wisechips-candle-light-oleds-installed-street-lights-aboriginal-korean-village; (b) http://www.oled-info.com/researchers-taiwan-urge-consumers-and-governments-watch-out-white-led-lighting ⁊
Jwo-Huei Jou is a professor in the Department of Materials Science & Engineering at National Tsing Hua University, Taiwan, and the president of the Chinese Organic Electronics Association. He can be reached at jjou@mx.nthu.edu.tw. Meenu Singh, Yi-Fang Tsai, Hui-Huan Yu, and Szu-Hao Chen are Ph.D. students from Prof. Jou’s group. Shang-Chih Lin is a Marketing Department Manager for WiseChip Semiconductor Inc. (www.wisechip.om.tw).