Developing Liquid-Crystal Functionalized Fabrics for Wearable Sensors
Developing Liquid-Crystal Functionalized Fabrics for Wearable Sensors
Textiles are the most universal wearable interface. Textile functionality is being rapidly expanded to incorporate a wide range of new technologies. A new concept of combining liquid crystals with fibers effectively incorporates all of the functionality associated with liquid crystals. In this article we report how liquid crystals can be incorporated into fibers and fabrics that respond visually to temperature. Looking to the future, we can envision how these liquid-crystal fabrics can be designed to respond to chemical and biological stimuli, signaling the presence of bacteria and viruses, and opening the potential for wearable medical sensors. In addition to the visual response, the fabrics can provide an electrical output, allowing them to be integrated into more sophisticated sensing systems.
by Junren Wang, Antal Jákli, Yu Guan, Shaohai Fu, and John West
In 1996, researchers at Georgia Tech prototyped the world’s first wearable motherboard1 or “intelligent” garment. This smart garment utilized optical fibers to detect bullet wounds, and special sensors and interconnects to monitor the body’s vital signs, with the aim of rescuing soldiers by monitoring their health status in real time. Recently, textile functionality has been expanded to incorporate a wide range of new technologies.2,3 Some wearable products, like Google Glass,4 the Apple Watch,5 and e-Tint eyewear6 are commercially available. Although “smart,” these products are not as flexible as fabrics and work differently from clothes.
The vast majority of synthetic fibers for textiles are manufactured using an extrusion process that involves pushing the melt or solution of the synthetic material through a spinneret to form filaments of the polymer or inorganic glass.7 The resulting fibers are used in a wide variety of applications, ranging from fabrics to complex fiber optic cables that make our communications network possible. Fiber-based devices and systems have outstanding flexibility, wearing comfort, and superior long-term fatigue resistance against large and repeated deformations. They are therefore well-suited for wearable applications.
As with many engineered materials, biology provides motivation and models for optimizing the fabrication and high performance of fibers. For example, spider silk is formed via a spinneret, utilizing fibroin proteins that produce a nematic liquid-crystal structure during the drawing process.8 The nematic alignment is essential to producing the high strength of the resulting fibers.9 Mimicking this naturally occurring process, Kevlar fibers10 were developed. These fibers have a highly ordered molecular structure and are produced using cold-spinning of a liquid-crystalline solution. Recent advancements in nanoscience and technology make it possible to replicate the complexity found in natural fibers and to add functionality not found in nature.
Wearable Sensors
We are particularly interested in forming wearable sensors. Sensors transform one type of signal into another. Different functional materials and structures have the capacity of transforming signals. These include color-changing materials,11 shape memory materials,12 and some bioinspired or biomimetic materials,13,14 just to mention a few. Thermotropic liquid crystals (discovered in 188815) are extremely useful for sensing due to their sensitive response to a variety of external stimuli. This is because they combine the fluidity of isotropic liquids with the anisotropy of crystals. Their large optical response to low voltages has led to their domination of today’s flat-panel display industry. The next generation of liquid-crystal devices is expected to continue exploiting this material’s sensitivity for signal transduction, but this time as wearable thermal,16 chemical,17 and biomedical18–20 sensors.
The color of chiral liquid crystals changes as their temperature varies. The color results from the spiral structure of the elongated molecules and the resulting periodic variation of the refractive index. The period of rotation, or pitch, is temperature-dependent and can be adjusted to coincide with the wavelength of visible light.
Liquid-Crystal Capsules
Thermochromic liquid crystals were first utilized about 50 years ago to sense temperature variations by spraying them directly on human skin.21 Later studies and patents in the 1970s reported using liquid-crystal thermography for evaluating inflammatory conditions22 and/or breast tumor detection.23,24 While technically effective, clinical application of liquid-crystal thermography was not successful. The procedure was often time consuming and messy, since it required either the patient’s skin to be painted black and then coated with an oily liquid-crystal film, or a flexible film to be stretched over the skin to display the temperature map.22 To eliminate the complications and messiness of prior art techniques, we
utilized thermochromic liquid-crystal capsules rather than unencapsulated and oily liquid-crystal materials.
The scanning electron microscope (SEM) image in Fig. 1 shows the morphology of commercially available thermochromic LC capsules fabricated by LCR Hallcrest.25 These spherical capsules are in sizes of several micrometers – several times smaller than the diameter of human hair. For each capsule, a thin and transparent polymer sheath encapsulates the thermochromic liquid crystal inside. These capsules [Fig. 2(a)] can also be embedded into fibers [Fig. 2(b)] by spinning a mixture of capsules and polymer solution.26 This represents a ruggedized approach, as the incorporated liquid-crystal capsules are locked into the flexible polymer fibers; therefore the fabric can be washed without losing the color response (Fig. 2).
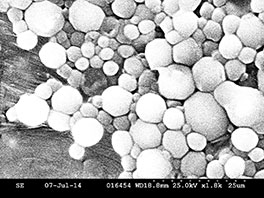
Fig. 1: A scanning electron microscope (SEM) image shows thermochromic liquid crystals in capsule form.
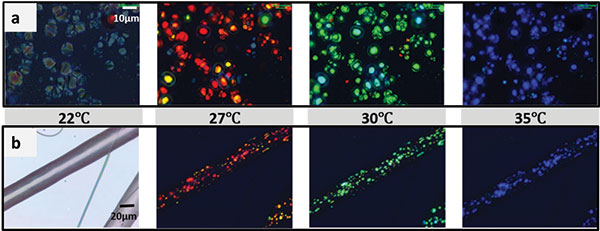
Fig. 2: (a) These reflected microscopic images of thermochromic liquid-crystal capsules result from different temperatures. (b) Fibers containing these capsules respond to varying temperatures by reflecting different colors.26
Liquid-Crystal Capsule Coating
With the protection of the sheath, these thermochromic capsules can be directly sprayed and/or printed onto the surface of an object to form a film. The impact of this reflected color is maximized when the chiral-nematic material is presented against a black background. For example, the capsules can be sprayed onto a black bandage to become a diagnostic medical tool to detect the arteries and veins in the wrist (Fig. 3).
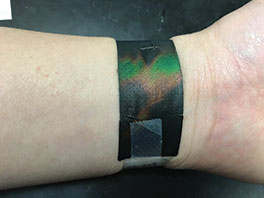
Fig. 3: Thermochromic capsules sprayed on a black bandage sense the arteries and veins located in the wrist area.
In order to achieve the required sensitivity, the color has to change over a narrow (1–2 °C) temperature range. Variations in average skin temperature among individuals and even in different circumstances in a single individual (such as mood) change the skin temperature and therefore can easily move out of the color response range of the selected chiral nematic mixture. Therefore, if the fabric only utilizes one chiral liquid-crystal formulation, it has the drawback of a narrow operating temperature range.
To allow the thermochromic fabric to have a high thermal sensitivity over a broad temperature range, we printed different patterns of multiple thermochromic capsule formulations whose visible thermochromic response occurs at different, adjacent, and complementary temperature ranges on a single fabric.27
Through simple paper stencils, these different mixtures are sprayed or printed into different patterns on the thermochromic fabric. The patterns provide another visual temperature reference that allows for high sensitivity and broad range. Figure 4 shows the thermal pattern of a human hand imaged on the fabric compared to a thermal camera image of the same hand.26 The fabric clearly shows how the printing of different chiral-nematic formulations in different patterns (a solid line, rectangles, and rhomboids) results in a sensitive thermochromic effect that operates over a broad temperature range. The same ensemble of thermochromic capsules can also be sprayed or printed on a tie to exhibit their fashion function.
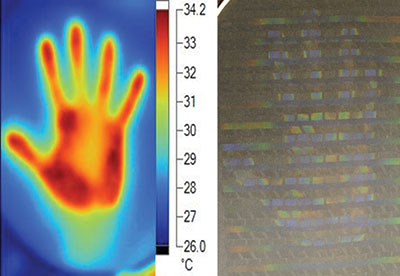
Fig. 4: Compare the thermal pattern of a human hand imaged on a thermochromic fabric (right) to a thermal camera image of the same hand (left).26
In this example, the color patterns of the tie change with changes in temperature, creating a chameleon effect (Fig. 5).26
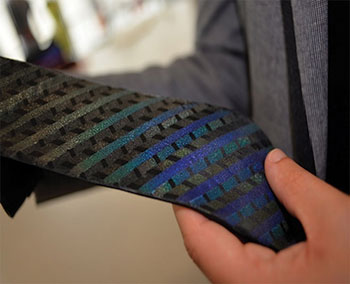
Fig. 5: In a fashion application, a tie is coated with thermochromic liquid-crystal capsules.26
Liquid Crystals/Polymer Cores/Sheath Fibers
Additionally, fluid liquid crystals can be embedded in fibers as a continuous liquid-crystal core surrounded by a polymer sheath. With the core/sheath structure, the polymer sheath protects the liquid crystals from harsh environments. The fiber structure and large surface-area-to-volume ratios place the liquid crystals in intimate contact with the external environment, increasing their sensitivity and response to changes in temperature28,29 and chemical vapors,30 etc. Direct incorporation of liquid crystal within polymer fibers was studied first
by Lagerwall, et al.,31 by using coaxial electrospinning, and then by West, et al.,29,32 by using single spinneret spinning and relying on spontaneous phase separation during the spinning process. By varying the working parameters during the spinning process,33,34 the morphologies of resulting fibers can be tuned between a beads-on-a-string structure and a uniform tubing structure (Fig. 6).
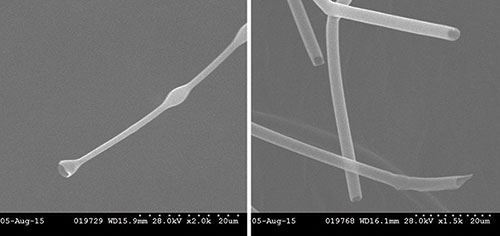
Fig. 6: These are examples of SEM images of liquid crystal/polymer fibers with different morphology – beads-on-a-string (left) and uniform core/sheath (right) fibers.
By spinning a solution containing a polymer and a temperature-sensitive chiral-nematic liquid crystal, non-woven liquid-crystal fiber mats can be obtained. The resulting fibers with different morphology show thermochromic response to changes in temperature as well (Fig. 7).
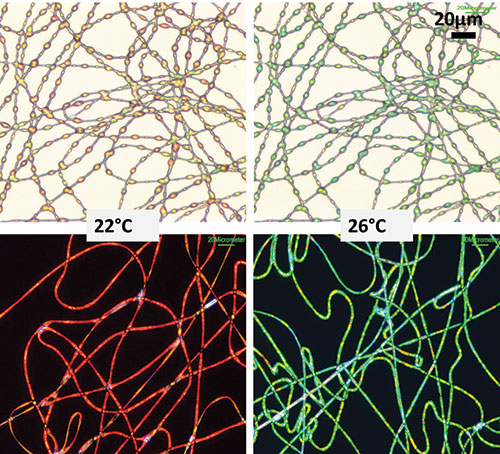
Fig. 7: Microscope images show how liquid-crystal fibers respond to temperature by reflecting different colors.
Instead of printing different thermochromic capsules in patterns on a black fabric, as shown in Fig. 4, we can fabricate complex fabrics by utilizing multi-spinning [Fig. 8(a)]. This produces fiber mats consisting of multiple fibers containing different
liquid-crystal compositions.26 The multi-jet electrospinning has been demonstrated as a 3D fabric printer (Electroloom35) and used to create a fabric for wearing. As shown in [Fig. 8(b)], using the rotating drum as a collector, the resulting fibers can be aligned and uniformly distributed. Importantly, the resulting hybrid fiber mats also possess enhanced capacity to respond to a variety of stimuli, since they contain different liquid crystals
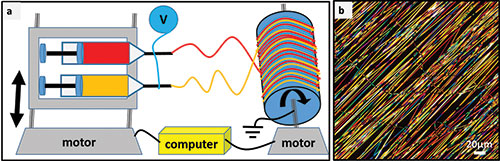
Fig. 8: In the multi-electrospinning set-up in (a), the spinnerets and rotating drum are moved or driven by a separate motor, which can be controlled by a computer. In (b) is shown a polarized microscopic image of aligned liquid-crystal/polymer fibers collected with the rotating drum.
Applications
We are at the infancy of the development of responsive liquid-crystal fibers. We anticipate many applications of responsive liquid-crystal fabrics and garments. The simple thermochromic fabric or garment may be worn on specific body parts of a patient. This may provide the first “killer” application as an effective medical diagnostic sensor that will move the technology from the laboratory to the marketplace. For example, a sock made from these fabrics could provide an early indication of foot ulcers in diabetic patients,36 a leading cause of complications and death from this increasingly common disease. Thermochromic fabrics could be fashioned into leggings worn by bedridden hospital patients, providing nurses and doctors with a quick, early warning of the development of a life-threatening thrombosis. As noted above, the thermochromic fabrics could be included in bandages to evaluate conditions of a developing infection and changes in blood circulation. As with the evolution of the LCD, these early, simple applications will undoubtedly be followed by increasingly sophisticated chemical and biological sensors that provide a range of visual, optical, and electrical outputs.
With Further R&D, the Potential Is Huge
To summarize, this article points to potential early applications of responsive liquid-crystal textiles for use as medical sensors. It predicts that these early applications will lead to more complex wearable sensors. We discussed and demonstrated that the next generation of liquid-crystal devices will be wearable and will exploit their sensitivity to thermal, chemical, and biological stimuli. This evolution requires a rethinking of how liquid-crystal devices are designed and fabricated. Incorporating liquid crystals into fibers, fabrics, and garments places the materials in contact with the external environment. Specifically, we reported the fabrication and performance of
thermochromic fabrics that incorporate liquid crystals, either as micro-capsules bonded to the fiber surface, or as coaxial fibers consisting of a liquid-crystal core surrounded by a polymer sheath. Such fibers and their assembly are as flexible and breathable as conventional fabrics.
Much research and development remain for our vision to become a reality. For example, the specific binding agents must be incorporated in the polymer sheath if we are to produce fibers that respond to specific chemicals or biological agents in the environment. We must also increase the durability of the fibers by crosslinking the polymer sheath and by utilizing thermally wide and chemically stable liquid-crystal formulations.
Acknowledgments
The authors acknowledge the support from Kent State University and Jiangsu Industrial Technology Research Institute (China), and acknowledge the scanning electron microscopy (SEM) characterization facility at Liquid Crystal Institute of Kent State University.
References
1www.smartshirt.gatech.edu
2B. J. Agarwal and S. Agarwal, “Integrated Performance Textiles Designed for Biomedical Applications,” IPCBEE 11, 114–119, 2011.
3A. Afif, et al., “An overview of smart technologies for clothing design and engineering,” Int. J. Cloth. Sci. Technol. 18, 108–128, 2006.
4www.google.com/glass/start
5www.apple.com/watch
6http://alphamicron.com
7Advanced Fiber Spinning Technology, Woodhead Publishing, 1994.
8F. Vollrath and D. P. Knight, “Liquid crystalline spinning of spider silk,” Nature 410, 541–548, 2001.
9D. P. Knight and F. Vollrath, “Spinning an elastic ribbon of spider silk,” Phil. Trans. R. Soc. Lond. B 357, 219–227, 2002.
10www.dupont.com/products-and-services/fabrics-fibers-nonwovens/fibers/brands/kevlar/products/dupont-kevlar-fiber.html
11M. Ferrara and M. Bengsu, Materials that Change Color: Smart Materials, Intelligent Design, Springer Science & Business Media, 2013.
12W. M. Huang, et al., “Shape memory materials,” Mater. Today 13, 54–61 (2010).
13N. Zhao, et al., “Bioinspired materials: From low to high dimensional structure,” Adv. Mater. 26, 6994–7017, 2014.
14M. Ebara, et al., Smart Biomaterials, Springer Japan, 2014.
15F. Reinitzer, “Beiträge zur Kenntniss des Cholesterins,” Monatshefte für Chemie und verwandte Teile anderer Wissenschaften 9, 421–441, 1888.
16L. Gao, et al., “Epidermal photonic devices for quantitative imaging of temperature and thermal
transport characteristics of the skin,” Nat. Commun. 5, 4938, 2014.
17C. G. Reyes, A. Sharma, and J. P. F. Lagerwall, “Non-electronic gas sensors from electrospun mats of liquid crystal core fibres
for detecting volatile organic compounds at room temperature,” Liq. Cryst. 43, 1986–2001, 2016.
18J. M. Brake, M. K. Daschner, Y. Luk, and N. L. Abbott, “Biomolecular Interactions at Phospholipid-Decorated Surfaces of Liquid Crystal,” Science 302, 2094–2097, 2003.
19S. Sivakumar, K. L. Wark, J. K. Gupta, N. L. Abbott, and F. Caruso, “Liquid crystal emulsions as the basis of biological sensors for the optical detection of bacteria and viruses,” Adv. Funct. Mater. 19, 2260–2265, 2009.
20S. J. Woltman, G. D. Jay, and G. P. Crawford, “Liquid-crystal materials find a new order in biomedical applications,” Nat. Mater. 6, 929–938, 2007.
21J. Fergason, Life Magazine, Jan. 18, 1968.
22M. Shlens, M. R. Stoltz, and A. Benjamin, “Orthopedic applications of liquid crystal thermography,” West. J. Med. 122, 367–70, 1975.
23E. Gitsch, “The value of liquid crystal thermography in the detection of breast cancer by the gynaecologist (author’s transl),” Wien. Klin. Wochenschr. 88, 737–740, 1976.
24R. Pochaczevsky and P. H. Meyers, “Vacuum contoured, liquid crystal, dynamic breast thermoangiography as an aid to
mammography in the detection of breast cancer,” Clin. Radiol. 30, 405–411, 1979.
25www.hallcrest.com.
26J. Wang, et al., “Smart Fabrics Functionalized by Liquid Crystals,” SID Symp. Digest of Tech. Papers 48, 2017.
27J. L. West, et al., “Thermochromic fabrics utilizing cholesteric liquid crystal material,” US patent 20150173679 A1, 2015.
28E. Enz and J. Lagerwall, “Electrospun microfibres with temperature sensitive iridescence from encapsulated cholesteric liquid crystal,” J. Mater. Chem. 20, 6866–6872, 2010.
29J. Wang, A. .Jákli, and J. L. West, “Airbrush Formation of Liquid Crystal/Polymer Fibers,” ChemPhysChem 16, 1839–1841, 2015.
30C. G. Reyes, A. Sharma, and J. P. F. Lagerwall, “Non-electronic gas sensors from electro-spun mats of liquid crystal core fibres for detecting volatile organic compounds at room temperature,” Liq. Cryst. 43, 2016.
31J. P. F. Lagerwall, J. T. McCann, E. Formo, G. Scalia, and Y. Xia, “Coaxial electrospinning of microfibres with liquid crystal in the core,” Chem. Commun. (Camb). 5420–5422, 2008.
32E. A. Buyuktanir, M. W. Frey, and J. L. West, “Self-assembled, optically responsive nematic liquid crystal/polymer core-shell fibers: Formation and characterization,” Polymer (Guildf) 51, 4823–4830, 2010.
33J. Wang, A. Jákli, and J. L. West, “Morphology Tuning of Electrospun Liquid Crystal/Polymer Fibers,” ChemPhysChem 17, 3080–3085, 2016.
34J. L. West, J. R. Wang, and A. Jákli, “Airbrushed Liquid Crystal/Polymer Fibers for Responsive Textiles,” Adv. Sci. Technol. 100, 43–49, 2016.
35www.kickstarter.com/projects/electroloom/electroloom-the-worlds-first-3d-fabric-printer.
36N. Singh, D. G. Armstrong, and B. A. Lipsky, “Preventing foot ulcers in patients with diabetes,” J. Am. Med. Assoc. 293, 217–228, 2005. •
Junren Wang, Antal Jákli, and John West are with the Liquid Crystal Institute at Kent State University. Wang and West are also affiliated with the Department of Chemistry and Biochemistry at Kent State and the Institute of Smart Liquid Crystal Technologies at JITRI. Yu Guan and Shaohai Fu are with the Engineering Research Center for Digital Textile Inkjet Printing at
Jiangnan University. West can be reached at jlwest@kent.edu.